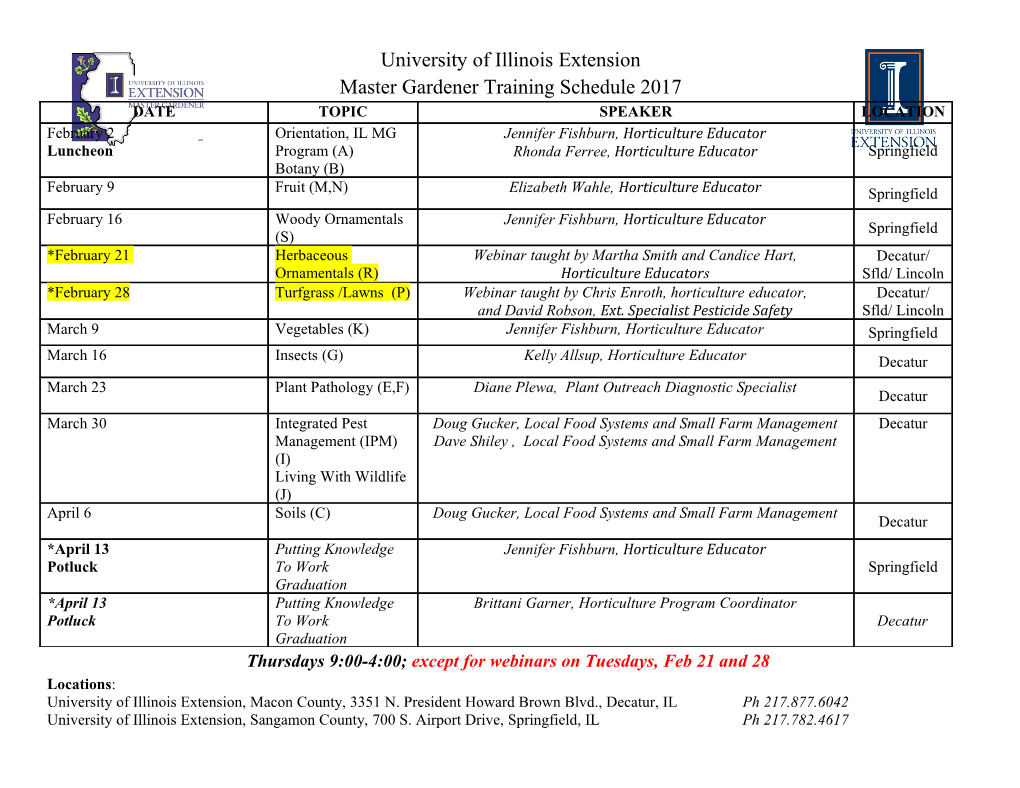
PROTOCOL Soft lithography for micro- and nanoscale patterning Dong Qin1, Younan Xia2 & George M Whitesides3 1Nano Research Facility and Department of Energy, Environmental, and Chemical Engineering and 2Department of Biomedical Engineering, Washington University, St. Louis, Missouri, USA. 3Department of Chemistry and Chemical Biology, Harvard University, Cambridge, Massachusetts, USA. Correspondence should be addressed to G.M.W. ([email protected]). Published online 18 February 2010; doi:10.1038/nprot.2009.234 This protocol provides an introduction to soft lithography—a collection of techniques based on printing, molding and embossing with an elastomeric stamp. Soft lithography provides access to three-dimensional and curved structures, tolerates a wide variety of otocols materials, generates well-defined and controllable surface chemistries, and is generally compatible with biological applications. It is also low in cost, experimentally convenient and has emerged as a technology useful for a number of applications that include cell biology, microfluidics, lab-on-a-chip, microelectromechanical systems and flexible electronics/photonics. As examples, here naturepr / we focus on three of the commonly used soft lithographic techniques: (i) microcontact printing of alkanethiols and proteins on m o gold-coated and glass substrates; (ii) replica molding for fabrication of microfluidic devices in poly(dimethyl siloxane), and of c . e r nanostructures in polyurethane or epoxy; and (iii) solvent-assisted micromolding of nanostructures in poly(methyl methacrylate). u t a n . w w INTRODUCTION w / / : The strategy of ‘smaller brings new capability’ has begun to change with an elastomeric stamp (Protocols for µCP, REM and SAMIM p t 1 t the world of biotechnology as it has transformed microelectronics . are described in the Procedure and outlined in Fig. 1). New variants h Successful applications of small systems include microarrays for such as dip-pen nanolithography have also emerged19–21. All these p 2 u high-speed DNA sequencing , microfluidic devices for perform- techniques use organic and polymeric materials that are referred o r ing PCR3, lab-on-a-chip (LOC) systems for synthesis and analysis G to as soft matter by physicists. 4 g of peptide and oligonucleotide libraries , and microchips for drug n i 5 6 h screening and for investigation of cultured cells . As the workhorse µCP s i 7 l of microfabrication, photolithography has contributed an impor- As the forerunner of soft lithography, µCP provides an attractive b u tant role in most of these applications, including the fabrication route to microscale patterns and structures needed for applications P e of DNA arrays in the late 1980s (ref. 8). However, this technique in biotechnology. For example, µCP offers an ability to engineer r u t has a number of limitations for applications related to biological the properties of a surface with molecular-level detail using self- a N systems. First, photolithography is an intrinsically expensive process assembled monolayers (SAMs) of alkanethiols on a substrate coated 0 because the equipment used was developed for the highly demand- with a metal such as gold (Au), silver (Ag), copper (Cu), palladium 1 0 22 2 ing processes required for fabrication of microelectronic devices. (Pd) and platinum (Pt) . In this study, the substrate refers to a © The capital investment required to build a clean room makes physical object, which is somewhat different from the meaning in photolithography less than accessible to most chemists, biochem- enzymology. Typical planar substrates for forming alkanethiolate ists and biologists. Thus, photolithography is most successful when SAMs include thin films of a metal deposited on silicon, mica, glass applied to a limited set of materials. Users wishing to study ‘dirty’ or even plastic materials. The substrates can be easily prepared by organic systems are usually excluded from an electronics-qualified physical vapor deposition methods, such as thermal or electron clean-room facility. Second, photolithography is often carried out beam evaporation23. The patterned SAMs have proved valuable for by projecting a pattern on a photomask onto a photoresist film. studying the role of spatial signaling in cell biology by exquisitely Although photomasks are commercially available, the time and controlling the molecular structure of a surface in contact with cost involved in the fabrication of such masks present a signi- cells24. One can create well-defined regions with ‘cell-friendly’ (protein- ficant barrier to the use of photolithography in rapid, inexpensive covered) and ‘cell-unfriendly’ (polyethylene glycol–terminated) SAMs prototyping of test patterns and devices. Third, photolithography to pattern endothelial cells on a surface and then examine how the provides little or no control over surface chemistry, and it is not cells respond to lateral confinement, and to being released from applicable to curved or non-planar substrates. confinement24. Furthermore, µCP has been successfully used to print Soft lithography9 represents a conceptually different approach to precise patterns of axon guidance molecules, which can then serve rapid prototyping of various types of both microscale and nano- as templates for growing chick retinal ganglion cell axons25. scale structures, and devices on planar, curved, flexible and soft substrates especially when low cost is required. A large number REM of patterning techniques—microcontact printing (µCP)10, replica Alternatively, REM provides a new method for fabricating micro- molding (REM)11, microtransfer molding12, micromolding in fluidic devices using poly(dimethyl siloxane) (PDMS)26, and this capillary13, solvent-assisted micromolding (SAMIM)14, phase- method has proved to be particularly suitable for various bio- shifting edge lithography15, nanotransfer printing16, decal transfer medical applications27–32. Compared with the inorganic materials lithography17 and nanoskiving18—form the basis of soft lithogra- such as silicon and glass that are used in microelectronics and phy; they are essentially based on printing, molding and embossing optics, and which have been explored but largely discarded for NATURE PROTOCOLS | VOL.5 NO.3 | 2010 | 491 PROTOCOL biologically directed microfluidics, PDMS offers a number of I. Design of pattern (Steps 1 and 2) unique and attractive features. They are33: (i) It has a shear modulus of 0.25 MPa and a Young’s modulus of roughly 0.5 MPa (charac- Photomask teristic of a moderately stiff elastomer). This elastomeric character II. Fabrication of master Positive resist Negative resist allows it to conform to a surface and achieve atomic-level contact, a (Steps 3–6) feature that is useful in forming and in sealing microfluidic systems. Substrate Substrate (ii) It is non-toxic and readily available from commercial sources at acceptable prices (~$80/kg). (iii) It is optically transparent down III. Fabrication of PDMS stamp to about 300 nm. (iv) It is intrinsically hydrophobic (with a water (Steps 6–11 or 12–19) contact angle of ~110°), but its surface can be modified by brief PDMS PDMS exposure to an oxygen plasma to become hydrophilic (with a water contact angle around 10°). (v) It can adhere and seal reversibly or, 26,34 IV. Soft lithography after oxidation, irreversibly to many different types of substrates . (Steps 20–46) The ease of production associated with PDMS microfluidic struc- otocols Microcontact printing of SAM Microcontact printing of protein tures will become even greater as the focus of microfluidics shifts (Steps 20–25) (Steps 26–29) from demonstration of components and devices to development PDMS PDMS of fully functional systems31,35,36. naturepr / Silicon Glass m Soft lithography also provides a set of attractive tools for nano- o c . fabrication. ‘Nanofabrication’ refers to a process that makes func- e r tional structures with at least one lateral dimension smaller than Silicon Glass u t a 100 nm (ref. 37). REM and SAMIM are two techniques based on n . w molding or embossing with an elastomeric stamp. Both can pro- Replica molding of PU or epoxy Solvent-assisted micro-molding of PMMA w (Steps 37–41) (Steps 42–47) w duce nanoscale structures in various soft materials, in parallel, over / / : 2 PDMS PDMS p large areas (3 cm with 100-nm features), and at relatively low cost. t t h When combined with selective etching and lift-off, these nanostruc- Solvent p tures have been transferred into metals and then used as substrates PU or epoxy PMMA u Substrate o Substrate r for biosensing. The methods of detection have included optical G 38 39 diffraction , surface plasmon resonance , surface-enhanced g 40 41 n Raman scattering and electrochemistry . i Substrate Substrate h s i l Figure 1 | Schematic illustration of the four major steps involved in soft b Photolithography versus soft lithography u lithography and three major soft lithographic techniques. P Photolithography will continue as the dominant technology in fabri- e r cation of semiconductor devices and systems that have stringent u t printing, molding and embossing. The term soft lithography ‘refers a requirements on the alignment, continuity, isolation and uniform- N 9 ity in the final patterns. There are, however, many existing and to the fabrication of patterned copies using the PDMS stamp’ ; 0 1 emerging applications for soft lithography that take advantage of and the overall process—from pattern design to fabrication of 0 2 (or require) the characteristics of these
Details
-
File Typepdf
-
Upload Time-
-
Content LanguagesEnglish
-
Upload UserAnonymous/Not logged-in
-
File Pages12 Page
-
File Size-