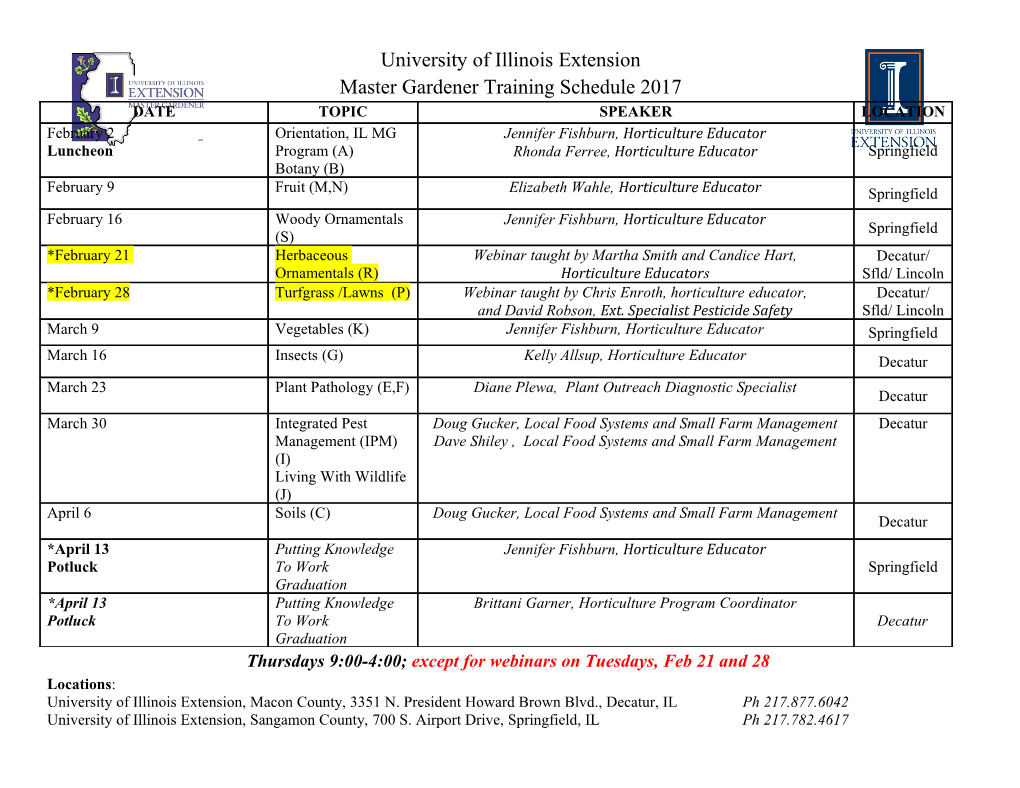
An Introduction to Grassmann Manifolds and their Matrix Representation Daniel Karrasch∗y Technische Universität München Center for Mathematics, M3 Boltzmannstr. 3 85748 Garching bei München, Germany September 26, 2017 In the following we give a self-contained introduction to Grassmann manifolds and their representation by matrix manifolds. The presentation is based on [6,1] and all results are taken from these references if not stated otherwise. Familiarity with calculus and elementary notions of differential geometry are assumed, for a textbook reference see, for instance, [12]. Throughout, let n 2 N>0 and k 2 f1; : : : ; ng. The object of investigation is the set of all k-dimensional linear subspaces V ⊆ Rn, i.e. Gr(k; Rn) := fV ⊆ Rn; V is a linear subspace; dim V = kg : The objective is to establish Gr(k; Rn) as a Riemannian (differentiable) manifold. 1 Topological & Metric Structure In this section, we follow [6]. We consider A 2 Rn×k as A = (aij)i2f1;:::;ng;j2f1;:::;kg = a1 ··· ak ; n n×k where ai = (aji)j2f1;:::;ng 2 R , i 2 f1; : : : ; kg. We endow R with the norm induced by the Frobenius inner product, i.e. q s > > X 2 kAk = tr(A A) = ja1j2 · · · jakj2 = aij: (1) 2 i;j ∗These notes were written while I was doing my PhD at Fachrichtung Mathematik, Technische Uni- versität Dresden, 01062 Dresden, Germany. Funding by ESF grant “Landesinnovationspromotion” No. 080942988 is gratefully acknowledged. yE-mail: [email protected] 1 We define the (non-compact) Stiefel manifold as n o St(k; Rn) := A 2 Rn×k; rk(A) = k n n×k o = A = a1 ··· ak 2 R ; a1; : : : ; ak are linearly independent : St(k; Rn) is often referred to as the set of all k-frames (of linearly independent vectors) in Rn. Note that St(k; Rn) is an open subset of Rn×k (as the preimage of the open set R n f0g under the continuous map A 7! det(A>A)). We define the compact Stiefel manifold as ∗ n n n > o St (k; R ) := A 2 St(k; R ); A A = Ik : p Clearly, St∗(k; Rn) is a bounded (by k) and closed (as the preimage of the closed set > n fIkg under the continuous map A 7! A A) and hence compact subset of St(k; R ) and St∗(k; Rn) ,! St(k; Rn). It is often referred to as the set of all orthonormal k-frames of linearly independent vectors in Rn. We consider the map n n π : St(k; R ) ! Gr(k; R );A = a1 ··· ak 7! span fa1; : : : ; akg ; (2) and endow Gr(k; Rn) with the final topology with respect to π, which we call the Grass- mann topology, i.e. U ⊆ Gr(k; Rn) is open if and only if π−1[U] is open in St(k; Rn). Let f 2 L(Rk; Rn) be injective and A 2 St(k; Rn) be the matrix representation with respect to the canonical bases in Rk and Rn. Then π(A) can be equivalently considered as f[Rk], i.e. the image of f. 1.1 Lemma. Consider Gr(k; Rn) with the Grassmann topology. (i) The map π is surjective and for any A 2 St(k; Rn) we have π−1[π(A)] = fAP ; P 2 GL(k; R)g : (3) (ii) The map π is continuous and open. Proof. Obviously, π is surjective and continuous. Furthermore, A; B 2 St(k; Rn) span the same subspace, i.e. π(A) = π(B) if and only if there exists a P 2 GL(k; R) such that B = AP . To see the sufficiency note that by assumption we have A = BP and B = AQ = BP Q for some P; Q 2 Rk×k, from which we directly read off that P = Q−1 2 GL(k; R). On the other hand, the necessity is obvious. For an open subset V ⊆ St(k; Rn) −1 S −1 we have π [π[V ]] = P 2GL(k;R)[V ]P , i.e. π [π[V ]] is a union of open subsets. Thus, π[V ] ⊆ Gr(k; Rn) is open by the definition of the Grassmann topology. Eq. (3) identifies Gr(k; Rn) with the quotient space St(k; Rn)=GL(k; R) := fA[GL(k; R)]; A 2 St(k; Rn)g ; (4) which can be endowed with the structure of a quotient manifold; see [2, Section 3.4]. However, we define a topological and differentiable structure on Gr(k; Rn) directly. 2 ∗ Rn : We consider the restriction of π to St (k; ), i.e. π = π St∗(k;Rn), and the “Gram- Schmidt orthonormalization map”, i.e. GS(A) is the matrix obtained by the application of the Gram-Schmidt orthonormalization method to the columns a1; : : : ; an of A. It is well-known that the Gram-Schmidt method defines a continuous map. Thus, we have π ◦ GS = π. Clearly, π is surjective and continuous. Next, we show a characterization of the continuity of functions f defined on a topo- logical space endowed with the final topology with respect to some other function and mapping to another topological space. 1.2 Lemma. Consider Gr(k; Rn) with the Grassmann topology. Let Ω be a topological space and f : Gr(k; Rn) ! Ω: Then the following statements are equivalent: (a) f is continuous; (b) f ◦ π is continuous; (c) f ◦ π is continuous. Proof. (a) , (b) follows from the definition of final topology with respect to π. (b) ) (c) is obvious since π is the restriction of π. (c) ) (b) follows from the representation f ◦ π = f ◦ π ◦ GS and the continuity of GS. One can show that the set ∆ := (A; B) 2 St(k; Rn)2; 9 P 2 GL(k; R): AP = B is closed in St(k; Rn) × St(k; Rn) by realizing that (A; B) 2 ∆ if and only if rk AB = k. This condition can be characterized by the requirement that all (con- tinuous) k + 1 minors of AB vanish. The set of matrices satisfying this condition is hence closed. Since Gr(k; Rn) = π[St∗(k; Rn)], π is continuous and St∗(k; Rn) compact, we have that Gr(k; Rn) is compact, too, and it remains to prove that the Grassmann topology is Hausdorff. To this end, let L; M 2 Gr(k; Rn) with L 6= M, A 2 π−1[fLg], B 2 π−1[fMg]. By Lemma 1.1 we have (A; B) 62 ∆ and since ∆ is closed, there exist neighborhoods UA and UB of A and B, respectively, such that (UA × UB) \ ∆ = ?. By Lemma 1.1 π[UA] and π[UB] are disjoint open sets containing π(A) = L and π(B) = M. Summarizing, we obtain the following result. 1.3 Proposition. Gr(k; Rn) is a compact space with respect to the Grassmann topology. Often, Gr(k; Rn) is endowed and investigated with the so-called gap metric, which we introduce next. 1.4 Definition (Gap metric). We define n n Θ: Gr(k; R ) × Gr(k; R ) ! R≥0; (L; M) 7! kΠL − ΠM k ; (5) where ΠX denotes the orthogonal projection on a subspace X and k·k denotes the oper- ator norm. Θ is called the gap metric on Gr(k; Rn). 3 In the literature, Θ(L; M) is also referred to as the opening between the subspaces L and M and was originally introduced in [11]. By the Projection Theorem for Hilbert spaces, there is a one-to-one correspondence between (closed) subspaces U and orthogonal ? projections ΠU with im ΠU = U and ker ΠU = U . Therefore, it is easy to see that Θ is indeed a metric on Gr(k; Rn). Next we show some further properties of the gap metric. 1.5 Proposition ([7, Theorems 13.1.1 & 13.1.2]). For L; M 2 Gr(k; Rn) (i) one has Θ(L; M) = max sup d(x; M); sup d(x; L) ; (6) x2S\L x2S\M where d(x; M) := inf fjx − yj ; y 2 Mg; (ii) Θ(L; M) = Θ(L?;M ?) ≤ 1; (iii) Θ(L; M) < 1 , L \ M ? = L? \ M = f0g , Rn = L ⊕ M ? = L? ⊕ M. Proof. (i) Let x 2 L \S. Then ΠM x 2 M and it follows jx − ΠM xj = j(ΠL − ΠM ) xj ≤ kΠL − ΠM k : Therefore, sup d(x; M) ≤ kΠL − ΠM k : x2S\L Analogously we have sup d(x; L) ≤ kΠL − ΠM k x2S\M and we obtain max sup d(x; M); sup d(x; L) ≤ kΠL − ΠM k = Θ(L; M): x2S\L x2S\M To derive the complementary inequality, observe that by the Projection Theorem in Hilbert spaces we have ρL := sup d(x; M) = sup jx − ΠM xj = sup j(I − ΠM )xj ; x2S\L x2S\L x2S\L ρM := sup d(x; L) = sup jx − ΠLxj = sup j(I − ΠL)xj : x2S\M x2S\M x2S\M Consequently, we have for every x 2 Rn that j(I − ΠL)ΠM xj ≤ ρM jΠM xj ; and j(I − ΠM )ΠLxj ≤ ρL jΠLxj : (7) We estimate 2 jΠM (I − ΠL)xj = hΠM (I − ΠL)x; ΠM (I − ΠL)xi = hΠM (I − ΠL)x; (I − ΠL)xi = hΠM (I − ΠL)x; (I − ΠL)(I − ΠL)xi = h(I − ΠL)ΠM (I − ΠL)x; (I − ΠL)xi ≤ j((I − ΠL)ΠM (I − ΠL)x)j j(I − ΠL)xj ; 4 thus by Eq. (7) 2 jΠM (I − ΠL)xj ≤ ρM jΠM (I − ΠL)xj j(I − ΠL)xj and hence jΠM (I − ΠL)xj ≤ ρM j(I − ΠL)xj : (8) On the other hand, using ΠM − ΠL = ΠM (I − ΠL) − (I − ΠM )ΠL and the orthogonality of ΠM , we obtain by Eqs.
Details
-
File Typepdf
-
Upload Time-
-
Content LanguagesEnglish
-
Upload UserAnonymous/Not logged-in
-
File Pages23 Page
-
File Size-