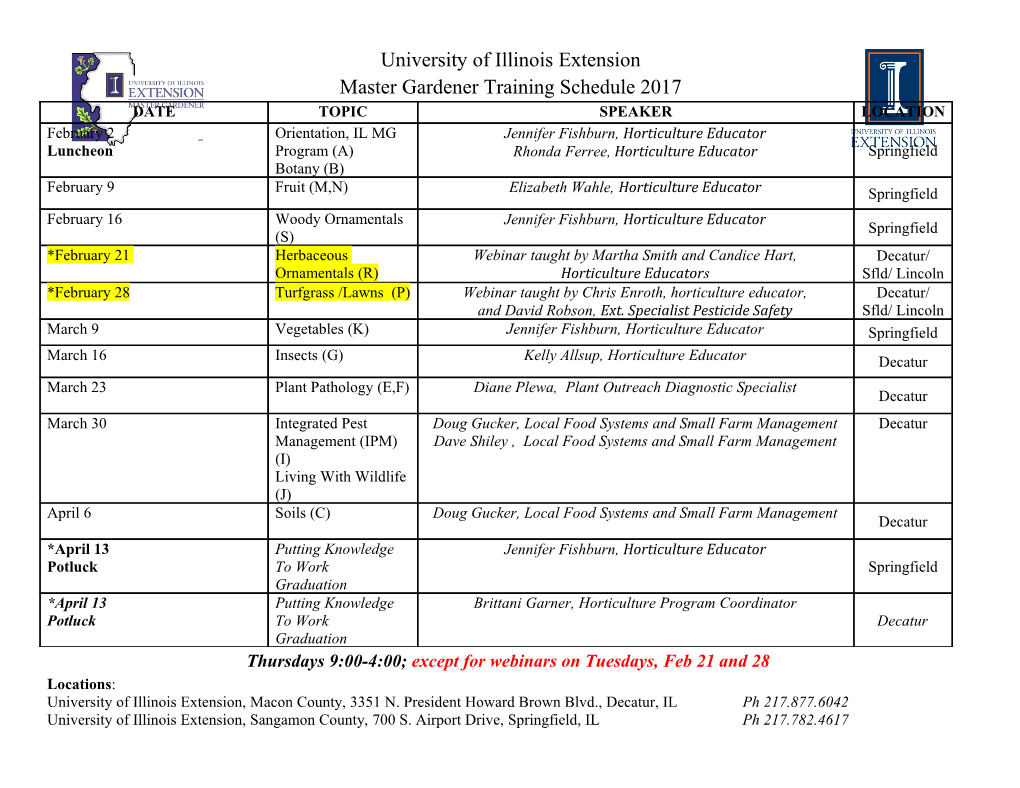
SnapShot: Force Spectroscopy and Single-Molecule Manipulation Yeonee Seol and Keir C. Neuman Laboratory of Molecular Biophysics, NHLBI, National Institutes of Health, Bethesda, MD 20892, USA TECHNIQUE GENERAL DESCRIPTION KEY CHARACTERISTICS APPLICATION EXAMPLES The deflection of a cantilever is measured High spatial resolution (0.5–1 nm); temporal resolution High force and high loading rate pull- Laser Photodiode using a laser beam and position-sensing (>1 KHz); high force range (10–104 pN); high loading rate ing and interaction assays; protein detector to obtain force and displacement (10–105 pN/nm). Low force resolution compared to OT; unfolding dynamics; protein-ligand for both imaging and force spectroscopy. In difficult to operate under constant force; specificity can interactions; ligand-receptor interac- force spectroscopy, the cantilever is attached be difficult to control. tions on live cells. to one end of the protein or DNA sample while the other end is immobilized on the surface. The force and position of the tip are measured as the surface is moved away from the tip, providing the force-extension curve Atomic force microscopy Atomic force of the sample. Single-beam gradient trap A high numerical aperture (NA) objective High spatial resolution (~1 nm) and temporal resolution Load-dependent enzyme activity focuses a laser to a diffraction limited spot, (10–50 kHz) if combined with laser detection system; of motor proteins such as kinesins creating an “optical spring.” Dielectric par- intermediate force range (0.1–100 pN). Photo damage on microtubules, myosins on actin. ticles (~µm sized beads, bacteria, organelles) and sample heating; difficult to maintain a constant DNA, RNA, and protein unfolding experience a restoring force toward the focus. force; sensitive to mechanical drift. and mechanical properties; DNA and Polystyrene or silica beads used as “handles” RNA polymerases; DNA and RNA attached to proteins or DNA permit precise hecliases on nucleic acids. measurements of force and displacement in addition to three-dimensional control over the position of the sample. Dual-beam (dumbbell) trap Two independent single-beam traps are Similar characteristics as single trap with potentially formed with one objective, and each end of higher spatial resolution due to insensitivity to surface the sample is held in one trap in a “dumbbell” drift; base-pair (<0.3 nm) spatial resolution. Photo dam- geometry. Force is applied by moving the age and sample heating; more complicated trapping position of one of the traps relative to the configuration. other. The traps can be moved in the speci- men plane by steering the trapping laser with movable mirrors, acoustic optic deflectors, or electro optic deflectors. Counter-propagating tweezers Two counterpropagating laser beams focused Similar characteristics with single trap; wider range of by two objectives enable a wide range of particles can be trapped. Similar drawbacks as single particles to be trapped with lower laser power trap; fixed optical trap position; complicated laser beam than other traps. A movable micropipet is alignment. typically used to manipulate the opposite end of the sample to impose the stretching force. Holographic OT (HOT) By imposing a spatially encoded phase on Parallel processing of multiple beads; rotation of opti- Cell sorting; mechanical manipulation Optical tweezers the trapping laser beam, arbitrary light pat- cally trapped objects; arbitrary trap configurations. of cell and lipid membranes. terns can be formed in the specimen plane. Photodamage and sample heating; difficult set-up and This permits arrays of hundreds of optical operations; slow reconfiguration (~10 Hz); decreased traps that can rotate asymmetric particles force and position resolution. and be moved or reconfigured under com- puter control. Optical torque wrench Torque can be applied to a trapped birefrin- High precision control of torque and force; high spatial DNA topology and mechanical prop- gent particle by rotating the polarization of and temporal resolution. Photodamage and sample erties of nucleosomes. the trapping laser. Birefringent particles that heating; difficult to maintain constant force; instrumen- have been used to apply torque in the optical tally challenging. torque wrench include µm-scale fabri- cated quartz cylinders and crushed vaterite particles. Dipole MT Two permanent magnets apply force and Magnetic tweezers (MT); spatial resolution (1–5nm); Manipulation of DNA topology. DNA Horizontal rotation on DNA attached to a superparamag- temporal resolution (~100 Hz); force range (0.01–300 topoisomerases and helicase assays. N S netic particle. The three-dimensional position pN); passive force clamp. Lower resolution compared to F o rce of the bead is obtained by video tracking. OT and AFM; no 3D trapping; no torque measurement. V e rtical The torque on the bead is sufficient in that it follows the rotation of the external magnets in a one-to-one correspondence. Rotor bead MT Attaching a reporter “rotor” bead at a nick Spatial resolution (1–5 nm); temporal resolution (~300 Torque-dependent DNA structural in the DNA allows rotation of the DNA to Hz); force range (0.01–100 pN); torque sensitive; angular transitions. Measurement of super- be directly observed. Force is applied on a resolution (~10°). Lower resolution compared to OT and coiling activity of DNA gyrase. superparamagnetic bead at the distal end of AFM; no 3D trapping. the DNA. Rotation and distortion of the DNA proximal to the rotor bead can be measured by the motion of the rotor bead. The torque on the DNA can be inferred from the rotation rate of the bead. Magnetic torque tweezers Using a cylindrical magnet with a small mag- Spatial resolution (1–10 nm), temporal resolution Torsional compliance of a RecA net attached on the side introduces a weak (0.1–0.01 s–1); force range (10–2–100 pN). Passive force nucleoprotein filament. S asymmetry in the circularly symmetric field clamp; torque sensitive; angular resolution (~0.1°). Low N along the axial direction that provides a small resolution compared to OT and AFM; no 3D trapping. torque and permits precise torque measure- Magnetic tweezers ments. Rotation of the bead is measured by tracking the position of a small nonmagnetic reporter bead attached to the magnetic bead. Single-pole MT (FOMT) Using a single magnet permits the applica- Spatial resolution (1–10 nm), temporal resolution Measurement of rotation by RNA S tion of force without torque. Torque can be (10–1~0.01 s–1), stiffness (10–3~10–6 pN/nm); force range polymerase transcribing DNA. Mea- N applied by using a shape-asymmetric particle (0.01–100 pN); passive force clamp; torque sensitive. surement of rotation associated with to break symmetry or by externally applying Low resolution compared to OT and AFM; no 3D polymerization of Rad51 on DNA. F o rce M rotating magnetic field using electromagnets. trapping; no rotational control. Rotation of the bead is measured by tracking the position of a small nonmagnetic reporter bead attached to the magnetic bead. 1168 Cell 153, May 23, 2013 ©2013 Elsevier Inc. DOI http://dx.doi.org/10.1016/j.cell.2013.04.047 See online version for legend and references. SnapShot: Force Spectroscopy and Single-Molecule Manipulation Yeonee Seol and Keir C. Neuman Laboratory of Molecular Biophysics, NHLBI, National Institutes of Health, Bethesda, MD 20892, USA Single-molecule manipulation and force spectroscopy have become indispensable biophysical tools, permitting manipulation and measurement of individual biomolecules at previously unimaginable levels of precision. In this SnapShot, we present three single-molecule force spectroscopy techniques widely employed in biological science: atomic force spectroscopy, optical trapping, and magnetic tweezers. Due to space limitations, we focused on these three techniques most commonly used for single-molecule manipu- lation. Further details can be found in recent reviews of individual techniques. ACKNOWLEDGMENTS This research was supported by the Intramural Research Programs of the National Heart, Lung, and Blood Institute, National Institutes of Health. REFERENCES Barroso, A., Woerdemann, M., Vollmer, A., von Bally, G., Kemper, B., and Denz, C. (2013). Three-dimensional exploration and mechano-biophysical analysis of the inner structure of living cells. Small 9, 885–893. Curtis, J.E., Koss, B.A., and Grier, D.G. (2002). Dynamic holographic optical tweezers. Opt. Commun. 207, 169–175. Inman, J., Forth, S., and Wang, M.D. (2010). Passive torque wrench and angular position detection using a single-beam optical trap. Opt. Lett. 35, 2949–2951. Lionnet, T., Allemand, J.F., Revyakin, A., Strick, T.R., Saleh, O.A., Bensimon, D., and Croquette, V. (2012). Single-molecule studies using magnetic traps. Cold Spring Harb. Protoc. 2012, 34–49. Lipfert, J., Hao, X., and Dekker, N.H. (2009). Quantitative modeling and optimization of magnetic tweezers. Biophys. J. 96, 5040–5049. Moffitt, J.R., Chemla, Y.R., Smith, S.B., and Bustamante, C. (2008). Recent advances in optical tweezers. Annu. Rev. Biochem. 77, 205–228. Müller, D.J., and Dufrêne, Y.F. (2008). Atomic force microscopy as a multifunctional molecular toolbox in nanobiotechnology. Nat. Nanotechnol. 3, 261–269. Neuman, K.C., and Block, S.M. (2004). Optical trapping. Rev. Sci. Instrum. 75, 2787–2809. Neuman, K.C., and Nagy, A. (2008). Single-molecule force spectroscopy: optical tweezers, magnetic tweezers and atomic force microscopy. Nat. Methods 5, 491–505. 1168.e1 Cell 153, May 23, 2013 ©2013 Elsevier Inc. DOI http://dx.doi.org/10.1016/j.cell.2013.04.047 .
Details
-
File Typepdf
-
Upload Time-
-
Content LanguagesEnglish
-
Upload UserAnonymous/Not logged-in
-
File Pages2 Page
-
File Size-