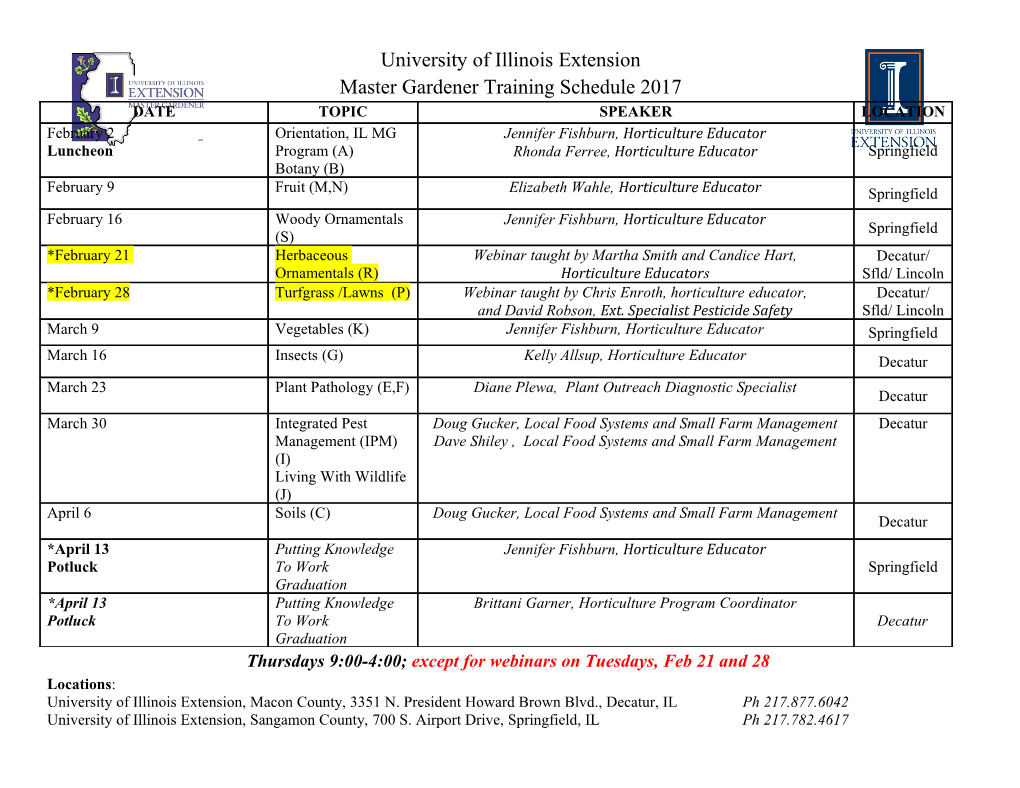
Advanced Synthesis and Catalysis ─ Hydrogenation and Hydrofunctionalization Chen Homogeneous vs. heterogeneous hydrogenation Heterogeneous catalysis occurs on catalyst surface. Substrate haptophilicity refers to the functional group affinity to catalyst Olefin hydrogenation is a thermodynamically favored process surface in directed hydrogenation. Blocking or poisoning active but the activation energy is high as the concerted addition of a sites reduces activity and increases selectivity. Metal cations, hydrogen molecule to an olefin is a symmetrically forbidden halides, sulfides, amines and phosphines are good poisons. reaction. However, transition metals can react with molecular hydrogen to generate metal hydrides that react with olefins Mechanistically, hydrogenation occurs with syn addition, but without violating symmetry rules. olefin isomerization on the catalyst surface leads to apparent anti-addition products. Isomerization usually occurs with Pd Hydrogenation can be catalyzed by either a homogenous or a but not Pt catalysts that bind to olefin irreversibly. Pt catalysts heterogeneous metal catalyst. In classic hydrogenation typically favor C=O over C=C reduction, especially when reactions, metal hydride is the key species that reacts with poisoned. Lindlar catalyst (Pd/CaCO3/PbO) has deactivated olefin. Monohydrides and dihydrides have different reactivities. surface that provides no over-reduction and cis/trans For example, HRh(PPh3)3(CO) is specific for terminal alkenes isomerization. The general order of reactivity is: and promotes olefin isomerization. In contrast, H2Rh(PPh3)2Cl has broader ,predictable olefin selectivities and does not Pt: C=O >> C=C > C-X > Ar promote olefin isomerization under neutral conditions. Pd: C=C > C-X > C=O > Ar Ru: C=O > C=C > Ar > C-X Homogenous catalyst is generally more reactive, selective for a single product, and easier to modify. However, catalyst poison Heterogeneous asymmetric hydrogenation can be realized with is a more serious issue and it is more difficult to separate from PdCl2 adsorbed on silk fibroin. Modification of Raney nickel the product. Therefore, most chemical processes use with chiral acids allows for asymmetric reduction of imines and heterogeneous catalysts. Homogenous catalysts are used ketones. However, the enantioselectivity is usually moderate. when the selectivity is critical and product-catalyst separation In contrast, there are many highly effective homogeneous problems can be solved. catalysts for asymmetric hydrogenation. 1 Advanced Synthesis and Catalysis ─ Hydrogenation and Hydrofunctionalization Chen Metal hydrides The hydride ligand normally occupies a normal coordination site, and the M-H bond distance is the sum of the covalent radii In classic hydrogenation, M-H is the active species that reacts of M and H. However, the hydride coordination site can be with olefins. Hydrogen ligands on transition metals, acidic or hard to find when the ligands are too big. For example, the hydridic, are always referred to as “hydrides”. The name of phosphine ligands of complex HRh(PPh3)4 take the four metal “hydrides” originates from the observation of upfield-shift corners of a tetrahedron. of hydrogen ligands in 1H NMR. However, the shift arises from the shielding effect of the partially filled d orbitals on the transition metal leading to mixing of low-lying electronic excited states into the ground state. The typical value for δM-H ranges from –5 to –25 ppm for d1 to d9 metal hydrides, but >0 ppm for 0 10 d and d metal hydrides. 6 HMn(CO)5 d δ –7.5 pKa 7.1 6 H2Fe(CO)4 d pKa 4.0 8 HCo(CO)4 d δ –10.7 pKa ≈ HCl, HNO3 8 HCo(CO)3P(OPh)3 d pKa 5.0 HMn(CO)5 8 HCo(CO)3PPh3 d pKa 7.0 0 H2ZrCp*2 d δ 7.46 HRh(PPh3)4 10 HCuP(p-Tol)3 d δ 3.50 Both the thermodynamic and kinetic acidity decrease going down the column of periodic table, with Cr>Mo>W, Fe>Ru>Os, but Pd<Ni<Pt, and increase from left to right across a row of the periodic table, with Zr-H and Ti-H being the most hydridic. In terms of the kinetic acidity, M-H is more similar to C-H (carbon acid) and the deprotonation rate is significantly slower than that of O-H or N-H. 1 Although the H chemical shift of HCo(CO)4 occurs at δ –10.7 in NMR, this metal “hydride” is highly acidic. It is somewhat weaker than HBr and H2SO4 and approximately equal to HCl H Re(PMePh ) and HNO . 5 2 3 3 2 Advanced Synthesis and Catalysis ─ Hydrogenation and Hydrofunctionalization Chen Hydride can also exist as a bridged ligand by forming a three- In addition to forming a µ2-H structure, the hydride can also center two-electron bond. Nearly all M(μ2-H)M structures adopt bridge multiple metal atoms to give µ3-H, µ4-H, µ5-H and µ6-H a non-linear geometry. As many as four bridging hydrides structures. between two metals have been observed. (μ3-H)Ru6(CO)17 (μ2-H)Ni2Br2(dippm)2 2– – [Cr2(CO)10] [(µ2-H)Cr2(CO)10] [H2Re(μ2-H)2(PEt2Ph)2]2 [(µ5-H)2Rh13(CO)24]32 – [(μ6-H)Co6(CO)15] 3 Advanced Synthesis and Catalysis ─ Hydrogenation and Hydrofunctionalization Chen Molecular hydrogen can also coordinate to metal centers The dissociation energy for M-H is generally 60 kcal/mol, through a σH-H→dσ orbital interaction. Backbonding of metal regardless of the natural of the metal or ligands. However, the center to the ligand though a dπ→σ*H-H orbital interaction dissociation energy of Os-H is greater than 78 kcal/mol. The further weakens the H-H bond. Groups 6–9 metals tend to interaction energy of Fe(μ-H)Fe is 81–85 kcal/mol. The –1 form the L-type M(H2) complexes whereas the more electron- stretching vibration of M-H in IR occurs at 2200–1600 cm rich group 10–12 metals having strong backbonding favor while that for bridging hydride occurs at 1600–800 cm–1. oxidative addition to form the X-type M(H)2 complexes. Metal hydrides can be generated from homolytic or heterolytic cleavage of H-H. Oxidative addition of hydrogen to metals gives metal hydrides with homolytic cleavage of H-H. Two- electron oxidative addition gives metal dihydrides while one- electron oxidative addition provides metal monohydrides. Elongation of the H-H distance upon coordination of H2 to a Hydrogenolysis of a M-M or M=M bond gives metal hydrides metal indicates a weakening of the H-H bond. The energy with one hydride per metal. barrier for moving the H-H distance from 1.00 Å to 1.60 Å, is only about 4 kcal/mol, readily tunable by ligands. H W(CO) (PiPr ) H OsCl(PPh ) 2 3 3 2 3 3 3 4 Advanced Synthesis and Catalysis ─ Hydrogenation and Hydrofunctionalization Chen Because oxidative addition requires a vacant coordination site, Heterolytic cleavage of H-H gives metal monohydrides without coordinatively saturated complexes usually react slower than changing the oxidation state of the metals. Same with oxidative coordinatively unsaturated complexes. addition, the metal center needs to have an empty orbital for 0 H2-binding. The only way that early transition metals with a d configuration react with hydrogen is through hydrogenolysis. Lanthanides and actinides also favor hydrogenolysis. The hydrogen ligand is acidified (ΔpKa up to 30 units) by the σ- binding of M-(H2). Metal hydrides can thus be produced by heterolytic cleavage of H-H through deprotonation with an + Reaction of Ir(cod)(PPh3) with 8-methylquinoline gave a external base. Protonation of the alkyl or halide ligand by the complex with an agostic C-H that non-dissociatively bound to Ir hydrogen ligand is referred to as hydrogenolysis. Conversely, via a 2-electron 3-centre C-H-Ir bridge. For 7,8-benzoquinoline, protonation of a basic metal hydride, e.g., HRe(CO)2(PMe2Ph)3 + the C-H bond is dissociatively bound to Ir giving a metal provides M-(H2) σ-complex (H2)Re(CO)2(PMe2Ph)3 . hydride. The water molecule of iridium complex H(H2O)Ir + (bq)(PPh3)2 is readily replaced by a hydrogen molecule. Based on HD isotope labeling experiments, there is also an exchange of the hydrogen atom between the Ir-H and Ir(H2) ligands through a trihydride intermediate. Heterolytic cleavage of H-H is favored over homolytic cleavage in polar environments commonly used for electrochemical or enzymatic reactions. Hydrogenase enzymes use bifunctional interaction to facilitate the heterolysis of H-H. 5 Advanced Synthesis and Catalysis ─ Hydrogenation and Hydrofunctionalization Chen The Wilkinson’s catalyst and Schrock–Osborn catalyst Wilkinson, Coffey, Vaska, and Bennett reported in 1965 the synthesis of the first practical hydrogenation catalyst Iguchi discovered in 1930s that catalytic hydrogenation can be Rh(PPh3)3Cl. Because of Wilkinson’s extensive work on its achieved with RhCl3, [Rh(NH3)5(H2O)]Cl3, or [Rh(NH3)4Cl2]Cl. catalytic activity, this complex is commonly known as Vaska reported in 1963 the synthesis of HRh(CO)(PPh3)3 and Wilkinson’s catalyst. Wilkinson studied in detail the activity of this monohydride. As a coordinatively saturated 18-electron complex, dissociation of Unconjugated olefins and acetylenes can rapidly hydrogenated a phosphine ligand is needed to generate the real catalyst. by Wilkinson’s catalyst in benzene. The use of a polar co- Because of the reversibility of the initial steps, internal olefins solvent facilitate the rate-limiting migratory insertion step. isomerize to terminal olefin without hydrogenation. Hydrogenation of terminal acetylene is faster than terminal olefin due to better binding and easier insertion especially when carried out with trifluoroethanol as a co-solvent. The reactivity of olefins negatively correlates with the steric hindrance for binding.
Details
-
File Typepdf
-
Upload Time-
-
Content LanguagesEnglish
-
Upload UserAnonymous/Not logged-in
-
File Pages30 Page
-
File Size-