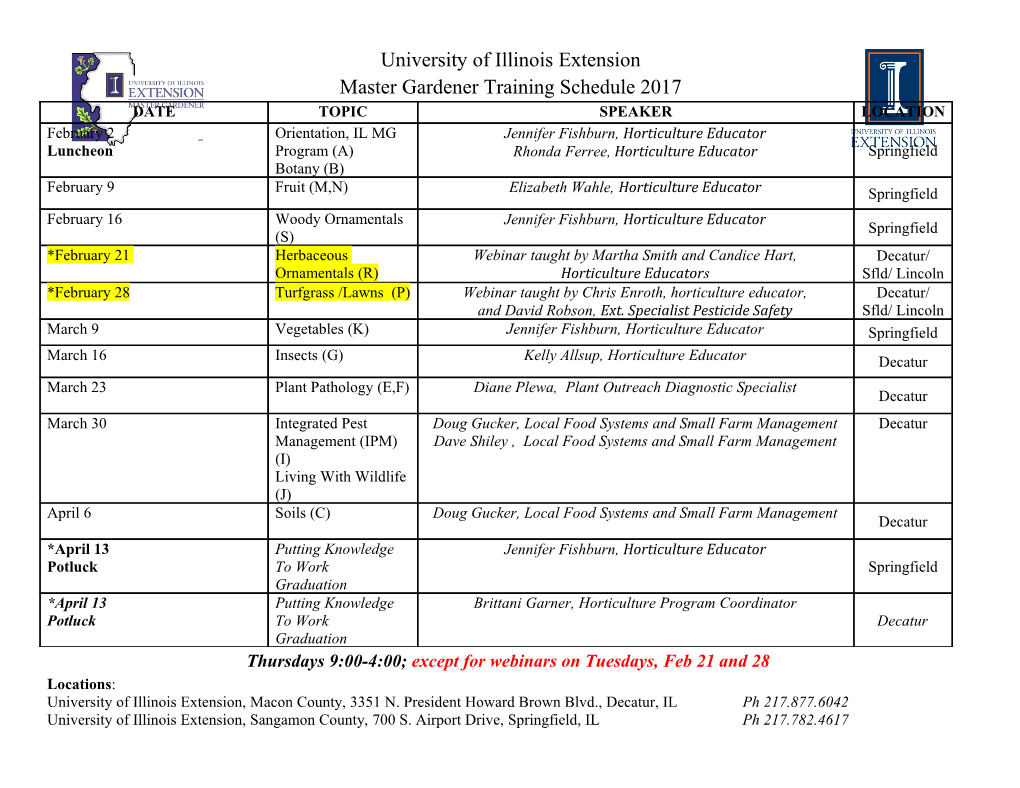
PHYSICAL REVIEW B 79, 115324 ͑2009͒ 4 Terahertz electromagnetic transitions observed within the I15Õ2 ground multiplet of Er3+ ions in Si S. Minissale,1 N. Q. Vinh,2 A. F. G. van der Meer,2 M. S. Bresler,3,* and T. Gregorkiewicz1 1Van der Waals-Zeeman Institute, University of Amsterdam, Valckenierstraat 65, NL-1018 XE Amsterdam, The Netherlands 2FOM Institute for Plasma Physics “Rijnhuizen,” NL-3430 BE Nieuwegein, The Netherlands 3A.F. Ioffe Physico-Technical Institute, RAS, 26 Polytekhnicheskaya, 194021 St. Petersburg, Russia ͑Received 23 January 2009; published 31 March 2009͒ An optically induced terahertz transition within the crystal-field-split ground state of Er3+ ion in Si has been conclusively established. A Si/Si:Er multinanolayer structure, where a single type of Er-related centers domi- nates, has been used. The study was conducted by pump-probe technique with a free-electron laser. Using the transient grating experimental configuration, we identify an absorption band at Ϸ43.5 m and measure the related effective lifetime as Ϸ50 ps. DOI: 10.1103/PhysRevB.79.115324 PACS number͑s͒: 78.55.Ap, 68.55.Ln, 78.47.JϪ, 78.67.Pt The 30–300 m wavelength range, which separates the be absent in Si ͑and Ge͒, which are nonpolar materials.6,7 predominantly optical and predominantly electric domains, is Consequently concepts of cascade lasers based on ͑strained͒ frequently referred to as the “terahertz gap” ͑1–10 THz͒. Si-Ge quantum wells have been put forward8,9 and their re- This reflects on the fact that the terahertz range remains alizations are being explored.10,11 poorly explored while almost the entire electromagnetic Rare-earth ͑RE͒ ions are frequently used for tailoring op- spectrum is exploited for practical applications, such as op- tical properties of insulating and semiconducting hosts. For tical communications and data storage, spectroscopy, elec- semiconductors, special attention has been given to Er dop- tronics and radiocommunications and telecommunications, ing of silicon, with the research clearly fuelled by prospect radar, and x-ray diagnostics among others. The main reason applications in Si photonics and optoelectronics—for a com- for this situation is that to date no cheap and practical prehensive review see, e.g., Ref. 12. RE-doped materials sources of terahertz radiation have been developed. In fun- have also been considered for generation of terahertz radia- damental research concerning, e.g., carrier dynamics, the tion. At a time, it appeared plausible to use for that purpose conveniently tunable free-electron lasers ͑FELs͒ remain the optical transitions between individual levels within a single most popular radiation source. These installations are, how- spin-orbit multiplet of a RE ion split by a local crystal field. ever, highly impractical in view of their size and operational This approach to terahertz generation turned out to be unsuc- costs. Another solution is offered by optically pumped mo- cessful due to large width, and therefore mutual overlap, of lecular lasers but these are also rather inconvenient with emission lines from individual crystal-field-split levels. The fixed emission wavelengths. At the same time, many inter- situation is considerably different in Si/Si:Er multinanolayer esting applications for terahertz range have been identified; structures grown by sublimation molecular beam epitaxy these include remote sensing ͑molecule identification͒ and ͑MBE͒,13 where a single type of Er-related optical centers is imaging for security and medical diagnostics, and also com- preferentially formed.14–16 The low-temperature photolumi- munications, where the terahertz band offers greater band- nescence ͑PL͒ spectrum of this material features a set of very width and reduced antenna sizes. Therefore research toward sharp lines with linewidth of ⌬EϽ8 eV. In this way, the development of a compact, versatile, and preferably electri- major obstacle hampering previous attempts of utilizing cally operated terahertz light source continues.1 Si:Er for development of a terahertz source has been re- In that context, a Si-based terahertz laser would be par- moved. In this study, we explore the possibility of optical ticularly welcome in view of the dominance of Si in contem- transitions between the well-defined crystal-field-split sub- 4 3+ porary electronics and the unprecedented sophistication of levels of the I15/2 ground state of the Er ion in a Si/Si:Er the Si complementary metal-oxide semiconductor ͑CMOS͒ multinanolayer structure. technology. Here the possibility to use transitions between The experiments have been performed on a Si:Er structure shallow donor and acceptor impurity states has been comprising 400 alternating Si and Si:Er layers—see Ref. 15 proposed,2 and stimulated emission from phosphor3 and for sample details. As mentioned before, Er3+ ions in this arsenic4 donors in Si has been demonstrated. However these sample are preferentially incorporated in a single type of solutions require separate and powerful optical pumping optically active center, designated Er-1, whose emission is ͑ ͒ FEL and CO2 laser in Refs. 3 and 4, respectively and cryo- characterized by ultranarrow emission lines. The ultrafast genic temperatures. Therefore their projected applications ͑picosecond resolution͒ pump-probe measurements in the are in space and atmospheric research rather than for the terahertz range have been performed at the Dutch free- terrestrial concepts mentioned earlier. An alternative solution electron laser user facility FELIX. All the measurements may be offered by quantum cascade lasers based on III-V were done at TՅ5 K with the sample placed in a helium gas compounds, which utilize intraband transitions,5 but opera- flow cryostat. The laser power incident on the sample was Ϸ ͓ tional temperature range of such devices is strongly ham- P 50 mW as measured at FEL=43 m, 1 GHz repetition pered by polar-optical phonon scattering. This effect should rate, and 7 s ͑macro͒ pulse duration͔. 1098-0121/2009/79͑11͒/115324͑5͒ 115324-1 ©2009 The American Physical Society MINISSALE et al. PHYSICAL REVIEW B 79, 115324 ͑2009͒ cm-1 6622.09 6554.82 4 I13/2 6502.85 τ =52ps 1 cm-1 232.89 τ =2ns 109.68 1 69.20 4 racted Beam Intensity I15/2 ff 59.12 Di 0 43 m FIG. 1. ͑Color online͒ Schematic of the energy-level scheme for 0 200 400 600 800 1000 the ground and the first-excited states of the Er-1 center. Gray zones Time (ps) indicate energy range where additional thus far unidentified levels should be located. Transitions observed in PL are shown by solid FIG. 2. ͑Color online͒ Result of the pump-probe measurement at arrows. Dotted arrows indicate possible terahertz transitions within FEL=43.5 m in transient grating configuration. A clear absorp- Ϸ the ground-state multiplet; in red ͑dark gray͒, the Ϸ43 m tran- tion band with a time constant of 1 52 ps can be seen, superim- ͑ Ϸ ͒ sition investigated in the current study is shown. posed on slowly decaying 2 2nsbackground, possibly of ther- mal origin. In the inset the interference pattern created on the In the past, we have precisely determined the crystal- sample by the split pump beam is shown. The fringe separation ⌳ field-induced splitting between sublevels of the ground state depends on the wavelength and the angle between the pump beams of the Er-1 center.15 This is illustrated in Fig. 1, where also :⌳=/2 sin͑/2͒. the most prominent PL transitions used to establish this en- ergetic scheme are indicated. On this basis, the wavelengths FTIR spectrum cannot be seen as a decisive argument of the possible terahertz transitions can be predicted. Identi- against the existence of the investigated transition. fication of the Ϸ43 m transition between the levels of Consequently, we performed pump-probe experiments in 4 3+ the I15/2 ground state of an Er ion—indicated in Fig. 1—is the =43 m range of the expected transition. In these mea- the main subject of this report. surements, an additional parameter appears—the lifetime of As an initial task, we carried out Fourier transform infra- the participating excited state. As mentioned before, for Er red ͑FTIR͒ absorption measurements on our sample in order we are dealing with a forbidden transition, while for B the to demonstrate the presence of a linear absorption band at the optical transitions are allowed. However in both cases re- Ϸ43 m wavelength of the predicted transition. Unfortu- combination transition probabilities are likely to be domi- nately, no absorption signal has been detected, while the re- nated by nonradiative processes, as phonon scattering will liability of the experiment was assured by observation of an prevail over photon emission. On the other hand, we can absorption signal of Ϸ30% and Ϸ65%, respectively, for the expect a considerable difference of the Huang-Rhys factor two boron-related transitions at 40.8 and 35.9 m. In order for B and Er, resulting in different coupling to lattice to explain the negative result, we note that only ϳ1–1.5 m phonons. The experiment has been performed in the tran- of the total thickness of the sample contains Er, with sient grating configuration. In this case, the FEL beam is a concentration of ͓Er͔=2ϫ1018 cm−3, while B is split into three parts by two polymer beam splitters ͑PP and 15 −3 ͒ ͑ ͒ present with a concentration of ͓B͔=2.7ϫ10 cm Mylar : two “pump” beams having wave vectors k1 and k2 in the ϳ500-m-thick substrate on which the Si/Si:Er and a “probe” beam, having wave vector k3—see inset of structure is grown. This implies that the areal density of Er Fig. 2. The two pump beams are spatially and temporally ͑ Ϸ ϫ 14 −2͒ NEr 3 10 cm is of the same order as that of B overlapped on the sample.
Details
-
File Typepdf
-
Upload Time-
-
Content LanguagesEnglish
-
Upload UserAnonymous/Not logged-in
-
File Pages5 Page
-
File Size-