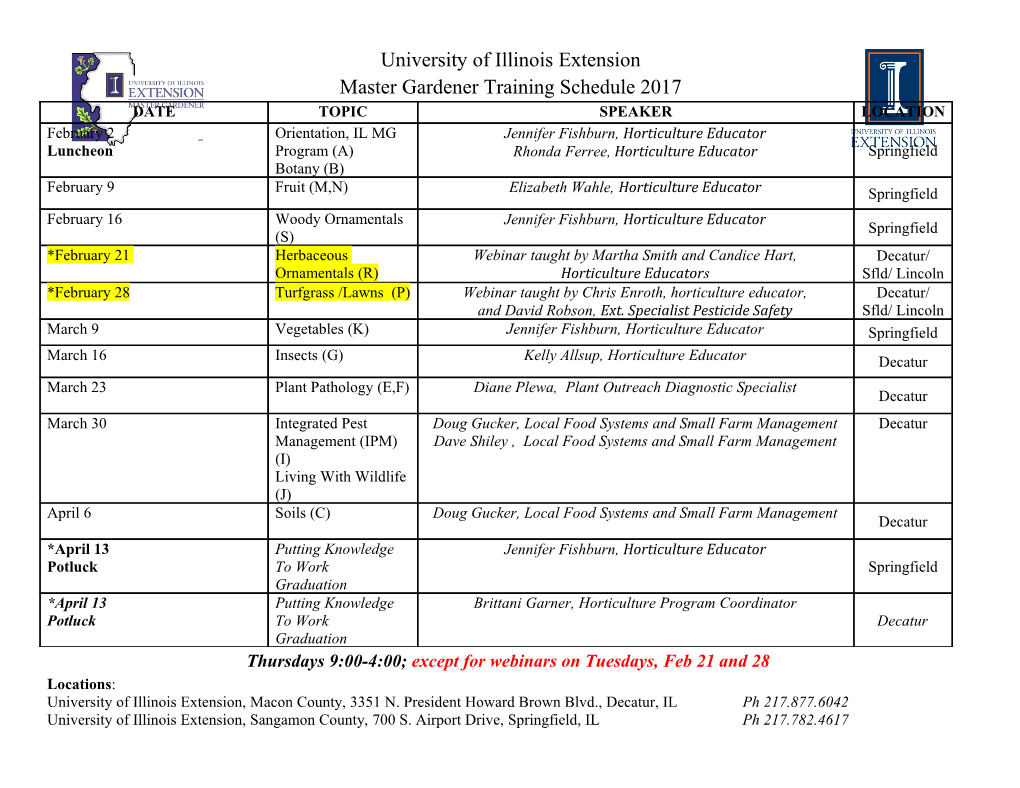
S0300-A8-HBK-010 CHAPTER1 NAVALARCHITECTURE FOR THE SALVAGE ENGINEER 1-1 INTRODUCTION Ships are built for a wide variety of purposes, but all must meet certain fundamental requirements. They must have reserve buoyancy to enable them to carry their designed loads and resist damage, stability to resist environmental forces or damage, and strength to withstand the stresses imposed on their structure by their own weight, cargo, stores, and the sea. The following discussion provides the salvage engineer with the basics of surface ship construction, stability, and strength. Submarine construction and stability are discussed in the U.S. Navy Ship Salvage Manual, Volume 4 (S0300-MAN-A6-040). Vessels are built to construction specifications based on stability and strength requirements, that are, in turn, based on intended service. Publicly owned vessels (Navy, Coast Guard, etc.) are built to government specifications. Most Navy ships are built to the General Specifications for Ships (GENSPECs), published by the Naval Sea Systems Command (NAVSEA), although some auxiliaries are built to commercial specifications. Stability standards for Navy ships are established by Design Data Sheet (DDS) 079 issued by the Naval Ship Engineering Center. Construction rules and stability standards for commercial vessels are established by classification societies, the International Maritime Organization (IMO), and government regulations for the country of registry; the American Bureau of Shipping (ABS) and United States Coast Guard (USCG) establish and enforce construction rules and stability standards for U.S. vessels. The U.S. rules are often based on IMO standards. The U.S. Maritime Administration (MARAD) may place additional requirements on ships built with Federal financial assistance. MARAD also produces standard designs for certain types of merchant ships. Stability and construction standards are discussed in Appendix C. There is a basic difference in the way naval architects and salvage engineers approach the problems of ship stability and strength. Naval architects, as designers, divide the subject into examinations of intact and damage conditions. The stability and strength of a proposed design is examined in normal operating, or intact, conditions, which must, as matter of course, include free liquid surfaces in tanks. Damage stability analysis examines a ship design in various hypothetical conditions of damage that include breaches in the immersed hull. The salvage engineer on the other hand, deals with damaged stability and strength, i.e., ships in conditions of known or identifiable damage, that may or may not include breaches in the immersed hull. There is a subtle distinction between damage and damaged stability. A salvage engineer doesn’t really deal with damage stability, or for that matter, with intact stability either. He deals with damaged stability, and conditions that can reasonably be attained from the initial damaged condition. While the salvage engineer also examines hypothetical conditions, those conditions usually have as a point of departure an initial damaged condition. This chapter discusses ship stability in light of those factors that provide and enhance stability, and those that impair or degrade. Those familiar with standard naval architecture texts may feel that this handbook’s treatment of the subject glosses over the distinction between intact and damage stability. This is true to some extent, because in the main, the distinction just doesn’t matter to salvage engineers; they deal with stability—good, bad, or indifferent—as they find it. The fact that free surface occurs in intact ships does not obscure the fact that it always impairs stability. 1-2 HULL FORM A ship’s hull is a complex geometric form that can be defined accurately by mapping its surface in a three-dimensional orthogonal coordinate system. If a Cartesian coordinate system is used, conventions usually set the Z-axis vertical, the X-axis longitudinal and the Y-axis athwartships. Principal dimensions are measured along these axes. The hull form can be shown in two dimensions by a series of curves formed by the intersection of the hull surface with planes parallel to these axes. The hull form, chosen by the designer, controls the stability and performance characteristics of the ship in its normal environments. 1-2.1 Location of Points Within a Ship. Because a ship is a three-dimensional mobile object, references within the ship itself must be established for locating points in, on, and about the ship. The position of any point in the ship can be described by measuring its position from reference planes or lines. The following planes are most commonly used: • Centerplane – A vertical plane passing fore and aft down the center of a ship; the plane of symmetry for most hull forms. • Design Waterplane – A horizontal plane at which the hull is designed to float. • Midship Plane – A transverse, vertical plane perpendicular to both the centerplane and the design waterplane, located at the midpoint of the molded hull length between perpendiculars on the design waterplane. • Baseplane – A horizontal plane passing through the intersection of the centerplane and the midships plane, or through the lowest point of the molded hull. 1-1 S0300-A8-HBK-010 The intersections of the reference planes with specified locations on the hull create additional reference lines and points: • Forward Perpendicular (FP) – A vertical line through the intersection of the stem and the design or load waterline (DWL, LWL). • After Perpendicular (AP) – A vertical line at or near the stern of the ship. In naval practice, the after perpendicular passes through the after extremity of the design waterline; in commercial practice, the after perpendicular usually passes through the rudder post, or the centerline of the rudder stock if there is no rudder post. • Midship Section (orMS) – An intersection of the midship plane with the molded hull. • Centerline (CL or CL) – The projection of the centerplane in plan or end views of the hull. • Baseline (BL or BL) – The projection of the baseplane in the side or end views of the hull. In ships with design drag where the baseline passes through the intersection of the midships section and the keel, parts of the hull will be below the baseline. For ships with flat-plate keels that float on an even keel, the baseline, bottom of the molded surface, and top of the keel plate coincide; if the keel plate is an outside strake (lapped over the adjacent strakes rather than butt-welded to them), the top of the flat-plate keel is below the bottom of the molded surface by the thickness of the strakes on each side of it (the garboard strakes). In vessels with hanging bar keels, the top of the keel coincides with the bottom of the molded surface. 1-2.2 Location of Points. The position of any point in the ship can be described by its: • Height above the baseplane or keel. • Athwartships position relative to the centerplane. • Longitudinal position relative to the midship section or to one of the perpendiculars. 1-2.3 Ship Dimensions. Molded dimensions, lines, etc., describe the fair surface defined by the framing and are principally of use to the shipbuilder. Displacement dimensions and lines describe the surfaces wetted by the sea and are of principal interest to the naval architect and salvage engineer in determining stability and performance characteristics. Extreme dimensions, such as extreme breadth, account for projections such as overhanging decks, fender rails, etc. Molded dimensions differ from displacement dimensions by the plating, planking, or sheathing thickness. In steel ships, this difference usually amounts to less than one percent of the total displacement. Displacement dimensions are not usually tabulated as such; if desired, they are deduced by adding plating thickness to molded dimensions, or deducting appendage measurements from extreme dimensions. The principal dimensions of a ship are length, beam, and depth. Two other important dimensions are draft and freeboard. Figure 1-1 shows the principal dimensions of a ship. • Length between perpendiculars (L, LBP or Lpp), is used for the calculation of hydrostatic properties. Length overall (LOA) is the maximum length of the vessel, including any extensions beyond the perpendiculars, such as overhanging sterns, raked stems, bulbous bows, etc. Length on the waterline (LWL or LWL) may or may not be the same as LBP, depending on the location of the perpendiculars; tabulated LWL is usually taken on the design waterline. • Beam or breadth (B) is the width of the ship. Molded beam is measured amidships or at the widest section from the inside surface of the shell plating. Maximum beam or extreme breadth is the breadth at the widest part of the ship, and is equal to the molded breadth plus twice the plating thickness plus the width of fenders, overhanging decks, or other solid projections. • Draft (T) is the vertical distance between the waterline and the deepest part of the ship at any point along the length. Drafts are usually measured to the keel and are given as draft forward (Tf), draft aft (Ta) and mean draft (T or Tm). A ship’s forward and after draft marks are seldom at the perpendiculars and mean draft is not necessarily amidships; the slight errors introduced by using drafts at these points can be discounted if trim is not extreme. Molded drafts are measured from the molded baseline, while keel drafts are measured from a horizontal line though the lowest point on the bottom of the keel extended to intersect the forward and after perpendiculars. Navigational or extreme drafts indicate the extreme depth of sonar domes, propellers, pit swords, or other appendages which extend below the keel, and are therefore not used to calculate hydrostatic properties. Draft scales for keel drafts are usually placed on both sides of the ship at each end as near as practical to the respective perpendiculars.
Details
-
File Typepdf
-
Upload Time-
-
Content LanguagesEnglish
-
Upload UserAnonymous/Not logged-in
-
File Pages109 Page
-
File Size-