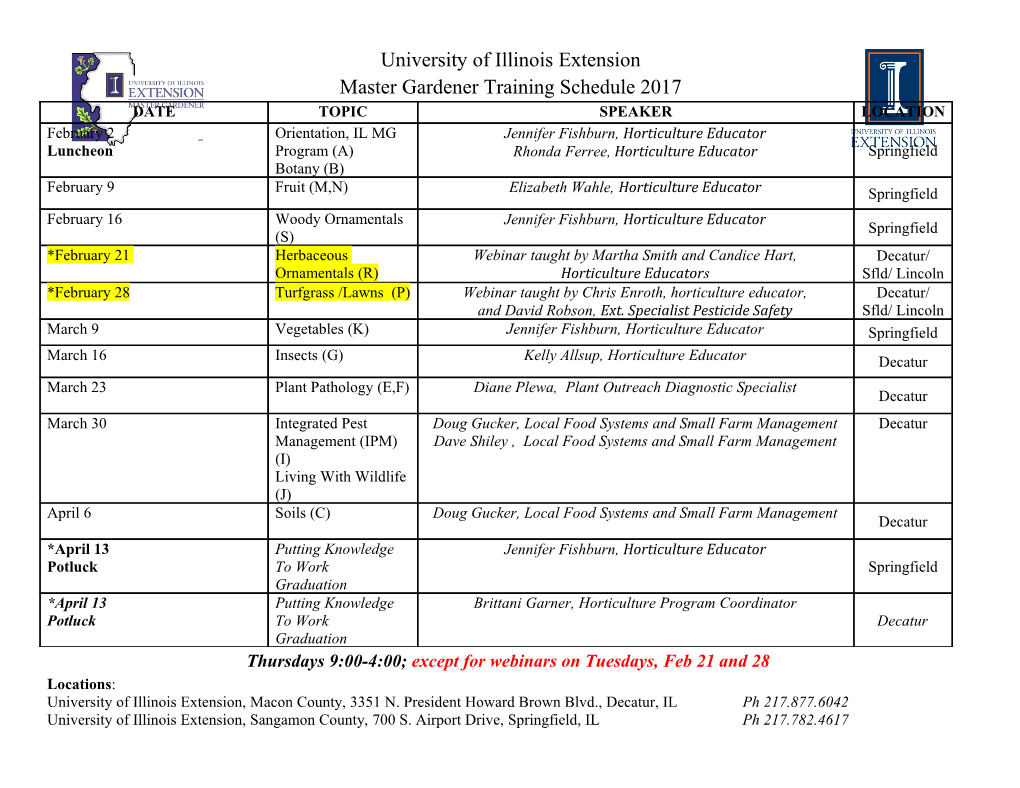
AWAKE: the proton-driven plasma wakefield accelerator experiment at CERN Erik Adli Department of Physics, University of Oslo, Norway on behalf of the AWAKE collaboration 6h International Conference on New Frontiers in Physics August 28, 2017, OAC, Crete, Greece AWAKE • AWAKE: Advanced Proton Driven Plasma Wakefield Acceleration Experiment – Use CERN SPS proton beam as drive beam (Single bunch 3e11 protons at 400 GeV) – Inject electron beam as witness beam • Proof-of-Principle Accelerator R&D experiment at CERN – First proton driven plasma wakefield experiment worldwide John Adams Institute for Accelerator Science, • AWAKE Collaboration: 18 institutes world-wide: Budker Institute of Nuclear Physics & Novosibirsk State University CERN Cockroft Institute DESY Heinrich Heine University, Düsseldorf Instituto Superior Tecnico Vancouver Novosibirsk Imperial College Ludwig Maximilian University Oslo Glasgow Max Planck Institute for Physics Manchester Hamburg, Greifswald Max Planck Institute for Plasma Physics London Dusseldorf, Marburg Rutherford Appleton Laboratory Munich TRIUMF CERN Lisbon University College London Ulsan Univesity of Oslo University of Strathclyde UNIST, Korea University of Marburg 2 Advanced accelerator research Research in new accelerator principles, with objective of overcoming accelerating gradient limitations of RF technology. Promise for more compact accelerators. Very high frequency normal conducting rf Dielectric structures structures (~100 GHz to ~THz) SiO2 ~ 1.0 THz, 116 GHz Accelerating structure fields of (SLAC) 300 MV/m demonstrated Laser based Plasma wakefield acceleration "DLA" acceleration Several 100 MV/m demonstrated The topic of this talk (SLAC) 3 Breakdown limits and plasma In metallic structures a too high field level will break down the surfaces, creating electric discharges. Field cannot be sustained. Structures may be damaged. Current practical limit (CLIC): order of 100 MV/m accelerating field. A plasma: collection of free positive and negative charges (ions and electrons). Material is already broken down. A plasma can therefore sustain very high fields. 4 Estimation of plasma wave field amplitude Assume that the plasma electrons are pushed out of a small volume of neutral plasma, with plasma density n0 = ne = nion : Scale of electrical fields (1D) : Gauss’ law: Plasma electron frequency : Assume wave solutions: Apply Gauss’ law: Field scale, EWB “wave breaking field” Example of neutral plasma density used for experiments : 3 n0 ~ 1e15/cm : EWB = 3 GV/m 5 Plasma wakefield acceleration (PWA) An intense particle beam, or intense laser beam, can be used to drive the plasma electrons. Focusing Decelerating Defocusing Accelerating - -- -------- ---- ------ -- ----- -- -+ + + -+--+ +--+ + + + --+ + +---+- + - + + e- Bunch -- ------ -- --- + + + -+-+++++++++++-+-+++++++ ++ ++-+-+ ++ ++ + + +-- + + + ++ -++---+- ++ ++ ++ ++-+--++ ++ ++ + + +- + + + + +--+- + + + + +- + + + + + + Laser Pulse - --+ + + + +--+---+- + + + -+-+----+ + + + + + - ---- - ----- -- ---- --- - -- v -~- -c---- -------- - Figure: Patric Muggli e- Witness - e Driver 14 20 3 15 3 Typical plasma densities used in experiment, 10 -10 /cm , For n0 ~ 10 /cm : • Field scale: EWB = 3 GV/m ~ √n0 -1 -1 • Length scale : kp =lpe/2p=1 mm ~ √n0 , plasma skin-depth kp =c / wpe • Radial focusing : Fr/c=½en0r/e0=30 kT/m Ideas of ~100 GV/m electric fields in plasma, using 1018 W/cm2 lasers: 1979 T.Tajima and J.M.Dawson (UCLA), Laser Electron Accelerator, Phys. Rev. Lett. 43, 267–270 (1979). Using partice beams as drivers: P. Chen et al. Phys. Rev. Lett. 54, 693–696 (1985) 6 Recent experiment progress in PWA Acceleration of beams to GeV-energies in plasmas has recently been demonstrated, using laser beams, electron beam and positron beams as drivers. Plasma wakefield accelerator M. Litos et al., (PWA) Plasma Phys. Control. Fusion 58 034017 (2016) GeV-energy gain in 1.3 m beam-driven PWA W. Leemans et al., Phys. Rev. Lett. 113, 245002 (2014) GeV-energy gain in 9 cm laser-driven PWA Challenge: towards TeV 7 Towards TeV beams While GeV acceleration in plasmas has been demonstrated for with both lasers and electron beams, reaching TeV scales requires staging of many drivers and plasma cells. Challenging. Plasma Plasma Plasma Plasma Plasma Plasma Witness beam cell cell cell cell cell cell Drive beam: electron/laser A possible witness beams: Existing driver beams options : Electrons: 1010 particles @ 1 TeV Lasers: up to 40 J/pulse ~few kJ Electron driver: up to 60 J/bunch Proton driver: SPS 19 kJ/bunch, LHC 300 kJ/bunch Proton drivers: large energy in proton bunches can consider single stage TeV acceleration: • A single SPS/LHC bunch could produce an ILC bunch in a single proton driven PWA stage. Plasma cell Witness beam Drive beam: protons + - p -Driver -1) e -Witness The wake of short proton drivers (~kp is - - - very similar to that of electrons drivers. -- - - --- - - -- - - - - ---- -- - + + + -+- - + +- - + + + + --+ + +-- - - - - ---- -- --- -+-+ + + + + + + + + + +-+-+ + + + + + + + + + +-+-+ + + + - + + + + -+---+- + + + +- -+ + +-+- ++ ++ + +++ ++-+- +-+ +++ ++++++++ ++-+-++ +++ - --- --- - -- + + + + +- +- - +- + + + -+ - +- -+ + - - - - -- -- - - - - - - -- - - - - --- - - - - 8 The self-modulation instability Kumar et al., PRL 104, 255003 (2010) radial fields lp Available proton bunches at CERN are 10 – 30 cm 14 3 long; lp for 7x10 /cm is 1.3 mm. Wakes will be weak; linear regime. The radial fields of the wake will modulate the beam density, creating microbunches spaced at the plasma wavelength. The evolution of the radius depends on the radius; self- modulation instability (SMI). The microbunches leads to resonsant build-up of strong Figure: Spencer Gessner wake fields (of order GV/m). 9 Seeded self-modulation instability The self-modulation can be seeded by a sharp start of the beam (or beam-plasma interaction). Will be done by laser ionization front co-propagating with proton beam. Short laser pulse No plasma Wake potential protons lp = 1.3 mm Accelerating fields for electrons 10 n cs tro ti ys os kl gn e, ia rc d ou n s tro ec el 0m 1 ll, ce Thea AWAKE experiment Use the sm la proton beam p ne strong wakefields400 GeV in a SPS li Location: CNGS tunnel am plasma. Acceleratem be oo n electron beamsr r to high ro se to drive ct energy. la ele at CERN ne -li - m er ea as b -l n on ng to ot gi ro pr er p m 750m proton beam line 11 fii f fif f fii fiiiff f fif ffiif i 198 E. Öz, P. Muggli / Nuclear Instruments and Methods in Physics Research A 740 (2014) 197–202 equivalent to providing uniform plasma density. The density uniformity of such a closed system can be estimated as follows: thedensity uctuationscan be related totemperature uctuations for an ideal gas with the ideal gas equation: p¼nkT where p is pressure, n is density and k¼1:38 10 23 m2 kg s 2 K 1 is the Boltzmann constant. At constant pressure, density and temperature uctuations are related by n T ¼ : n T The uctuations result from two sources: the externally imposed Fig. 2. (a) Sketch of the plasma source. Two independently heated sections consist of a10 m long Rb vapor section with fast valves for proton, electron and laser beam and internal uctuations. The internal uctuations are estimated accessand valved Rb liquid reservoirs. (b) Photo of thevalved Rb liquid reservoir by from theaveragevalueof temperature uctuationsfor an ideal gas MBEKomponenten incorporated. [3] at temperature Twhich is given by kT2 purchased from MBE-Komponenten incorporated [5] Fig. 2(b). 〈ð TÞ2〉 ¼ cV They contain only a few grams of Rb. An advantage of having twoindependent heatingsystemsfor thereservoirsand thepipeis where c is the heat capacity at constant volume. This can be put V that by keeping the temperature of the pipe a few degrees above in a useful form to calculate the temperature uniformityf i i fi f that of the reservoirs prevents condensation of Rb in a cold spot s ffiffiffiffiff ffi s ffiffiffi f f f f 〈ð TÞ2〉 k inside the pipe, all the condensation occurs in the reservoir. 2 ¼ 5 0:002: Rubidium is known to form dimers and clusters at these T cV densities [6]. However, the number of dimers or clusters is Thereforetheexternally imposed uctuationsdominateand hence expected to be small when compared to the number of single a density uniformity of n=nr 0:002 can be achieved with a atoms. In addition, a fraction of non-atomic species will not effect temperature uniformity of T=Tr 0:002. the plasma density uniformity unless they are themselves non- uniformly distributed in this constant temperature environment. Several methods are described in literature to get rid of these 2. Description of Rb vapor source dimers[7], such as by super heating the vapor or by using adiode laser tuned across Rb 2 D2 resonance line. It is also possible that Rubidium is chosen because of its low ionization potential after thepassageof thebeamstheclusterization may be enhanced (URb ¼4:177 eV), and relatively low temperature requirement to by plasmaelectronsseeding [8]. Sincethereservoir and thesource obtain the desired vapor density when compared to other alkali can be independently heated and isolated it ispossibletoget rid of metals. Rubidium has a melting temperature of 38.89 1C, conse- non-atomic species by dumping the vapor onto a cold plate at quently it is in solid form at room temperature. The temperature room temperature and completely re ll the source from the range in order to reach the required densities is 150–200 1C. i reservoirs between events. This is determined by the vapor pressurffi e curve
Details
-
File Typepdf
-
Upload Time-
-
Content LanguagesEnglish
-
Upload UserAnonymous/Not logged-in
-
File Pages33 Page
-
File Size-