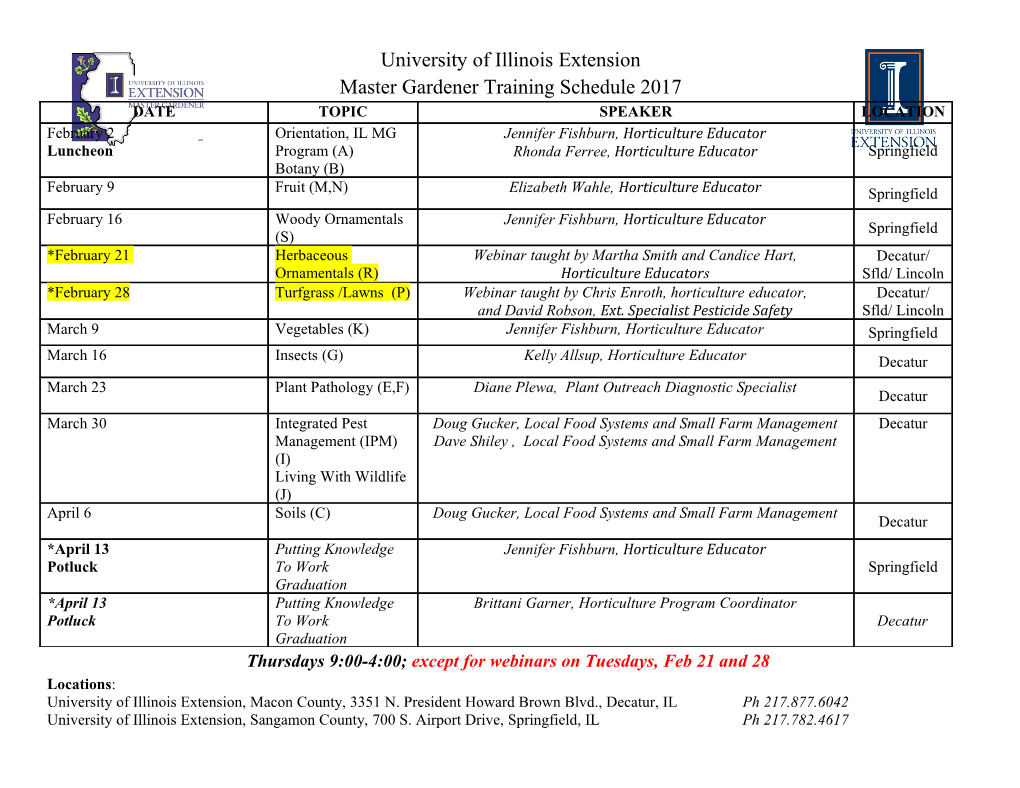
*Manuscript Click here to view linked References 1 2 TEM-HRTEM study on the dehydration process of nanostructured Mg-Ca 3 4 5 hydroxide into Mg-Ca oxide 6 7 8 L.S. Gomez-Villalba1*, A. Sierra-Fernandez1,2 , M.E. Rabanal2,3 R.Fort1 9 10 11 1 Instituto de Geociencias (CSIC, UCM), Calle José Antonio Novais 12, 28040 Madrid, Spain 12 13 14 2 Materials Science and Engineering Department Universidad Carlos III de Madrid, Avda. 15 16 17 Universidad 30, 28911 Leganés, Madrid, Spain 18 19 20 3 Instituto Tecnológico de Química y Materiales Alvaro Alonso Barba (IAAB) , Avda. 21 22 Universidad 30, 28911 Leganés, Madrid, Spain 23 24 25 26 KEYWORDS: Nanoparticles, crystal growth, defects, HRTEM, EELS, SAED, radiolysis. Brucite, 27 28 periclase 29 30 31 * Corresponding author: Luz Stella Gomez-Villalba [email protected], 32 33 34 [email protected] 35 36 37 38 Abstract 39 40 41 The dehydration process from Mg 0.97Ca0.03 (OH)2 nanoparticles (brucite type hexagonal 42 43 44 structure) to Mg0.97Ca0.03 O (periclase type cubic structure) was studied by Transmission 45 46 Electron Microscopy (TEM-HRTEM), Electron Diffraction (SAED), EELS spectroscopy (EELS) and 47 48 image analysis. The transformation process was monitored in function of the reaction time 49 50 51 applying 200 and 300 KV. Changes in porosity were possible to observe only during the 52 53 irradiation with 200 KV. Depending on the irradiation time, the changes were gradual, 54 55 producing an increase from the particle´s edge towards the inner region. Different stages were 56 57 58 observed, corresponding to the amount of water extracted from the particle, until finally a 59 60 decrease in porosity and particle shrinkage occurs, coinciding with the formation of the Mg-Ca 61 62 1 63 64 65 oxide. However, when samples were exposed to 300KV, the dehydration process was much 1 2 faster, and the pores structure was destroyed in a shorter time in comparison with lower doses 3 4 of radiation. High resolution electron microscopy (HRTEM) applying 300kV allowed identifying 5 6 7 the progressive changes from brucite to periclase, including the formation of an intermediate 8 9 dehydrated phase. The transformation along [0001]brucite and [10¯10]brucite orientations was 10 11 12 monitored determining differences in the kinetic of reaction related to the presence of point 13 14 defects affecting the atomic lattice. 15 16 17 Key words: nanoparticles; electron irradiation; phase transformation; brucite; periclase; Mg-Ca 18 19 20 Hydroxide; Mg-Ca Oxide 21 22 23 24 1. Introduction 25 26 27 The irradiation of nanomaterials with electrons shows a great variety of fundamental importance 28 29 phenomena that may be studied by in-situ electron microscopy. Modern TEMs offer the possibility to 30 31 study materials with resolution down to 0.1 nanometres or even less, enabling the understanding of 32 33 radiation effects at atomic scale. An advantage of in-situ electron microscopy is that structural 34 35 36 transformations can be induced and imaged with the same electron beam and studied in real time with 37 38 atomic resolution[1]. It has often been observed that a system relaxes from a metastable to the 39 40 equilibrium state under the electron beam[1].This is the case of porous solids, when used as catalyst or 41 42 catalyst supports, that are frequently produced by dehydration of hydroxides. These materials show 43 44 their importance in all applications due to their wide internal surface area on which adsorption and 45 46 47 reaction can take place. The electron microscope technique not only enables the observation of these 48 49 catalysts pore structure directly, but also the dehydration reactions to be followed in situ [2, 3]. In 50 51 inorganic materials, both knock-on and radiolysis may take place during their exposition to the electron 52 53 beam, sometimes simultaneously. However, the radiolysis process has been observed predominantly in 54 55 insulators (halides, oxides, hydrides, hydroxides, sulphides and silicates) while knock-on in conducting 56 57 materials [4]. Radiation alters the physical properties of materials such as electro- conductivity, 58 59 60 deformation, mechanical properties, etc [5]. During the radiation, vacancies and interstitial atoms are 61 62 2 63 64 65 grouped to form secondary defects such as clusters, dislocation loops and pores [5]. Although High- 1 2 Resolution Transmission Electron Microscopy (HRTEM) is primarily a technique for studying bulk defects, 3 4 it has been applied in the profile-imaging mode to derive information about surfaces [6]. Nowadays the 5 6 EELS spectroscopy provides excellent alternatives to monitor the time evolution of chemical reactions 7 8 [7, 8 ]. 9 10 11 12 Nowadays with the improvement in resolution, it is possible to observe the complex nanostructure of 13 14 catalytic materials. There is no other experimental technique able to provide such a localized and direct 15 16 view of both surface and bulk microstructure [9]. Spectroscopic measurements performed in situ within 17 18 the microscope provide elemental analysis and give information on oxidation state and bonding [10].The 19 20 decomposition reactions represent an important class of solid state reactions, thus the understanding of 21 22 their mechanism as well as kinetics is extremely important for synthesis of desired materials [11]. 23 24 25 Most catalysts based on simple or mixed oxides are often derived from the dehydration of hydroxides. 26 27 28 In the case of magnesium hydroxide, the dehydration technique is suitable for experimental 29 30 investigation because of the existence of only two well-crystallized phases during the process, brucite 31 32 (Mg(OH)2) and periclase MgO [12, 13], according to the following reaction: 33 34 35 Mg(OH)2 (brucite) (s) → MgO (periclase) (s) + H2O (g) 36 37 38 Brucite structure is representative of a group of bivalent metal hydroxides M(OH)2 where M= Mg, Ca, 39 40 Mn, Fe, Co, Ni or Cd with a compact hexagonal packing[14]. The structures are layered, where cations 41 42 are placed in the octahedral interstices between two sheets of (OH)- arranged parallel to (0001) and 43 44 every (OH) - is linked to three cations[15]. This structure is also common in other minerals, as brittle 45 46 47 micas which are built up of alternate mica- and brucite-like layers [16]. The dehydration shows some 48 49 common patterns of behaviour as the dehydroxylation processes of simple hydroxides (Mg(OH)2, 50 51 Ca(OH)2 [17]. 52 53 54 The reaction mechanism has been thoroughly studied in literature due to its wide application in 55 56 decomposition of other hydroxides through a combination of different complementary experimental 57 58 approaches [18,19]. In thermal dehydration, a simple Mg(OH)2 crystal (P3m1) with CdI2 type structure 59 60 61 62 3 63 64 65 is converted into a NaCl-type MgO cubic crystal (Fm3m)[20], as a result of a topotactic reaction[21]. 1 2 Previous studies of differential thermal analysis (DTA), thermogravimetry (TG) or in situ X Ray Diffraction 3 4 [22,23,24]have permitted to delimit the transformation temperature between 300 and 350°C, in 5 6 Mg(OH)2 nanoparticles obtained from different synthesis methods. On the other hand, Mg(OH)2 could 7 8 also be dehydrated by radiating with high-energy electrons[12,13,19,21,25]. During a TEM session, the 9 10 local temperature reaches values between 500 and 600°C [26]. Nowadays, the inclusion of a heating 11 12 13 stage that is capable of rapidly heating the sample with minimal thermal drift has allowed to see high 14 15 resolution images obtaining important advances in dynamic high resolution transmission electron 16 17 microscopy during in situ Environmental-TEM experiments [27]. With this tool has been possible to 18 19 understand the mechanisms of sintering in nanoscale particles [27] including the MgO by Mg(OH)2 20 21 dehydration in a short time[28]. Thus, the transmission electron microscopy techniques result very 22 23 useful for the understanding of the kinetics of brucite into periclase transformation. 24 25 26 Magnesium hydroxide has proven to be of great interest in medicine, industry, in environmental 27 28 29 rehabilitation and recently in the conservation of cultural heritage [29], due to its outstanding physical 30 31 and chemical properties. Brucite is an industrial mineral with an excellent market-growth potential. It 32 33 also represents a potential ore source for the metal, magnesium, which is itself in high demand [30]. 34 35 Such brucite and periclase can be used as catalysts [31], refractory [32], thermal insulator [33], fire 36 37 retardant [34], or in steel coatings[35]. Also, brucite and periclase are used as reinforcement in 38 39 nanoparticles for biomedical applications [36] and as anti-acid in the pharmaceutical industry [37].Other 40 41 42 applications include the use of Mg(OH)2 for chemical heat storage in nuclear reactors [38,39]. Hence, 43 44 tracking the process of dehydration by the electron beam is quite useful in the field of energy 45 46 technology. 47 48 49 Catalysts containing two or more metals might show significantly different catalytic properties 50 51 compared with the parent metals and thus might be widely used in several catalytic reactions [40,41]. 52 53 There are many similarities between pure Mg hydroxide with Mg- Ca hydroxide, although the presence 54 55 of calcium within the Mg structure could modify its behaviour. Dehydration process could have large 56 57 58 implications when calcium is incorporated for obtaining Mg-Ca hydroxide, instead of having pure Mg 59 60 hydroxide.
Details
-
File Typepdf
-
Upload Time-
-
Content LanguagesEnglish
-
Upload UserAnonymous/Not logged-in
-
File Pages33 Page
-
File Size-