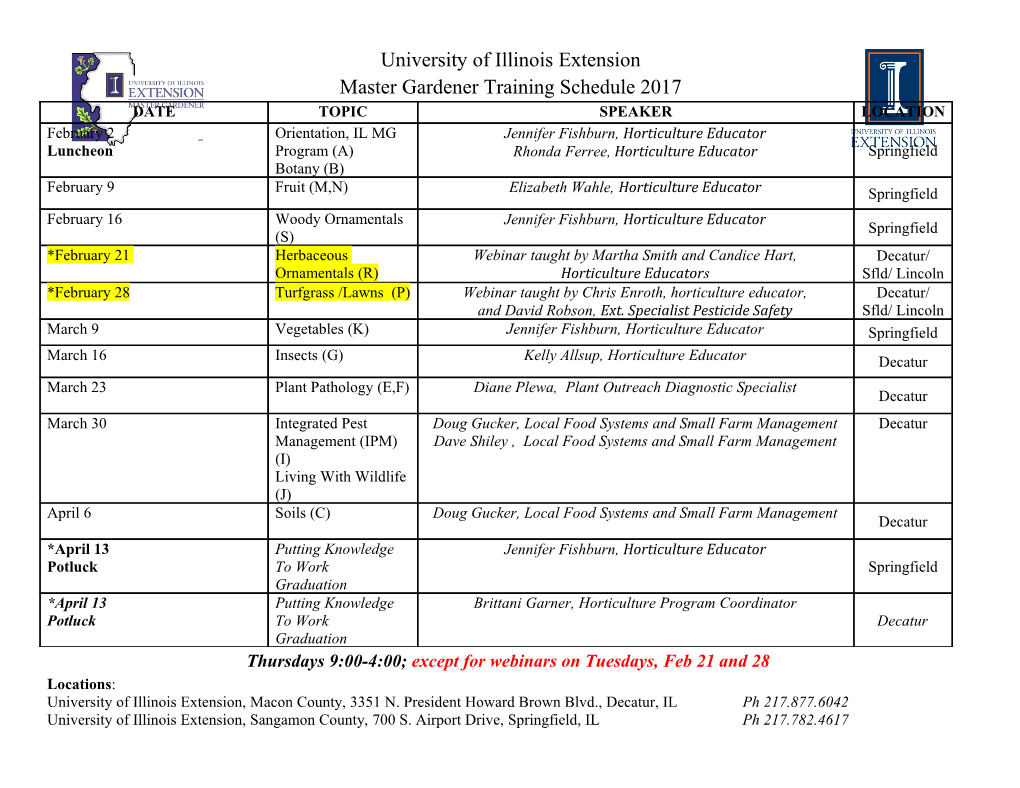
This is a repository copy of Dynamic Antarctic ice sheet during the early to mid-Miocene.. White Rose Research Online URL for this paper: http://eprints.whiterose.ac.uk/96977/ Version: Accepted Version Article: Gasson, E., DeConto, R.M., Pollard, D. et al. (1 more author) (2016) Dynamic Antarctic ice sheet during the early to mid-Miocene. Proceedings of the National Academy of Sciences, 113 (13). https://doi.org/10.1073/pnas.1516130113 Reuse Unless indicated otherwise, fulltext items are protected by copyright with all rights reserved. The copyright exception in section 29 of the Copyright, Designs and Patents Act 1988 allows the making of a single copy solely for the purpose of non-commercial research or private study within the limits of fair dealing. The publisher or other rights-holder may allow further reproduction and re-use of this version - refer to the White Rose Research Online record for this item. Where records identify the publisher as the copyright holder, users can verify any specific terms of use on the publisher’s website. Takedown If you consider content in White Rose Research Online to be in breach of UK law, please notify us by emailing [email protected] including the URL of the record and the reason for the withdrawal request. [email protected] https://eprints.whiterose.ac.uk/ 1 69 2 A dynamic Antarctic Ice Sheet during the early to 70 3 71 4 middle Miocene 72 5 73 6 Edward Gasson1, Robert DeConto1, David Pollard2, Richard Levy3 74 7 75 8 1Climate System Research Center, University of Massachusetts, Amherst, MA, 01003, USA 2Earth and Environmental Systems Institute, Pennsylvania State 76 9 University, State College, PA, 16802, USA 3GNS Science, Avalon, Lower Hutt 5011, New Zealand 77 10 78 Submitted to Proceedings of the National Academy of Sciences of the United States of America 11 79 12 Geological data indicate that there were major variations in preindustrial levels [1518] to maximum concentrations of only 80 13 Antarctic ice sheet volume and extent during the early to middle 500 ppm [15,16,18,19]. 81 14 Miocene. Simulating such large-scale changes is problematic be- Simulating such large-scale variability of the Antarctic ice 82 15 cause of a strong hysteresis effect, which results in stable ice sheets sheet with this narrow range of atmospheric CO2 has proved 83 16 once they have reached continental size. A relatively narrow range problematic [20,21]. This is due to a strong hysteresis, which limits 84 17 of atmospheric CO2 concentrations indicated by proxy records retreat from a fully glaciated state until surface temperatures 85 18 exacerbates this problem. Here we are able to simulate large-scale have increased by 1520 C [22] or atmospheric CO2 has reached 86 19 variability of the Miocene Antarctic ice sheet for the first time due 10002500 ppm [20, 23]; see also Supporting Information. This 87 20 to three developments in our modeling approach: 1. We use a new hysteresis occurs because of surface elevation-mass balance feed- 88 21 climate-ice sheet coupling method using a high-resolution atmo- back as a result of the atmospheric lapse rate [24], and is further 89 22 spheric component, to account for ice sheet-climate feedbacks 2. strengthened by albedo feedback [20] and possibly the cooling 90 23 The ice sheet model includes recently proposed mechanisms for effect of the ice sheet on the surrounding Southern Ocean [56]. 91 24 retreat into deep subglacial basins caused by ice-cliff failure and Recent efforts at resolving the Antarctic hysteresis problem 92 25 ice-shelf hydrofracture 3. We account for changes in the oxygen have focused on the marine-based regions of the ice sheets 93 26 isotopic composition ofSubmission the ice sheet by using isotope enabled PDF 94 [23,25]. This work has been stimulated in part by evidence for 27 climate and ice sheet models. We compare our modeling results major retreat into the Wilkes Subglacial Basin during warmer 95 28 with new ice-proximal records emerging from a sedimentological intervals of the middle Pliocene [26] and the need to explain 96 29 drill core from the Ross Sea (Andrill-2A) that is presented in the, albeit uncertain, 20 ±10 m middle Pliocene sea level high- 97 30 a companion paper. The variability in Antarctic ice volume we stand [27,28]. Additionally, the marine-based ice sheet regions 98 31 simulate is equivalent to a seawater oxygen isotope signal of are thought to be more sensitive to climate changes than the 99 32 0.520.66 , or a sea level equivalent change of 3036 m, for a terrestrial-based regions because of instability mechanisms that 100 33 range of atmospheric CO2 between 280500 ppm and a changing act only on marine-based ice, particularly where the ice sits 101 34 astronomical configuration. This result represents a substantial on reverse-sloped beds [23,29,30]. However, only a third of the 102 35 advance in resolving the long-standing model-data conflict of modern Antarctic ice sheet is marine-based [31], which suggests 103 36 Miocene Antarctic ice sheet and sea level variability. that the variability during the Miocene also included changes in 104 37 terrestrial ice sheet extent. 105 38 Miocene | Antarctic ice sheet | oxygen isotopes | sea level Evidence of grounded ice in the western Ross Sea during 106 39 the Miocene provides another modeling challenge. Simulating a 107 40 Both direct and indirect evidence indicates that the Antarctic large, grounded West Antarctic ice sheet typically requires Last 108 41 ice sheet exhibited major variation in volume and extent during Glacial Maximum-like conditions, with low atmospheric CO2 109 42 the early to middle Miocene (2314 million years ago, Ma). of ∼180 ppm, cold ocean temperatures, and lowered sea level 110 43 Indirect evidence for a change in Antarctic ice volume comes 111 44 18 112 from O records of benthic foraminifera [15] and sea-level 45 113 indicators 6 8]. Although the benthic 18O record contains a Significance 46 [ 114 mixed signal of ice volume and ocean temperature, attempts to 47 115 isolate the ice volume component show variability equivalent to Atmospheric concentrations of carbon dioxide are projected 48 116 the loss of between 30% 2,3] and 80% 4,5,9] of the modern to exceed 500 ppm in the coming decades. It is likely that 49 [ [ the last time such levels of atmospheric CO were reached 117 Antarctic ice sheet. A similar magnitude of variability is indicated 2 50 was during the Miocene, for which there is geologic data 118 by sea level estimates 6 8]. This may represent periods of ice 51 [ for large-scale advance and retreat of the Antarctic ice sheet. 119 advance with volumes greater than modern and periods of retreat This is something that ice sheet models have struggled to 52 120 with significant (but not complete) loss of ice. replicate because of a strong hysteresis effect. Here a number 53 of developments in our modeling approach mean that we are 121 54 Direct evidence of the Miocene Antarctic environment can able to simulate large-scale variability of the Miocene Antarctic 122 55 be found in ocean sediments drilled at sites proximal to the ice ice sheet for the first time. Our results are also consistent with 123 sheet, such as the Andrill 2A (AND-2A) drill core in the southern a recently recovered sedimentological record from the Ross Sea 56 presented in a companion paper. 124 57 McMurdo Sound region of the Ross Sea (see companion paper). 125 58 These records show warmer conditions in the Ross Sea region Reserved for Publication Footnotes 126 59 during the middle Miocene climatic optimum, with summer at- 127 60 mospheric temperatures of 10 C and annual mean sea-surface 128 61 temperatures between 011.5 C [10,11]. During these warmer 129 62 intervals the ice sheet margins retreated inland [12,13] and tundra 130 63 vegetation grew on ice-free terrain [10,14]. During cold intervals 131 64 the ice sheet expanded, with grounded ice extending into the Ross 132 65 Sea basins, beyond the AND-2A drill site [12]. 133 66 Importantly, proxy reconstructions of atmospheric CO2 con- 134 67 centrations through this interval imply that this variability oc- 135 68 curred in a relatively narrow range from close to, or slightly below, 136 www.pnas.org --- --- PNAS Issue Date Volume Issue Number 1--?? 137 removed from the continent can be loosely constrained by vol- 205 138 umes of Oligocene and younger sediments deposited offshore 206 139 of the continent; Wilson et al. [34] used this as a basis for their 207 140 topographic reconstruction for the Eocene-Oligocene transition. 208 141 As, to our knowledge, no reconstructed topography for the 209 142 Miocene currently exists, we created a middle Miocene topogra- 210 143 phy by scaling between the earlier Eocene-Oligocene topography 211 144 [34] and the isostatically rebounded (ice-free) modern topogra- 212 145 phy [41], assuming constant rates of landscape evolution from 213 146 34 Ma to today. An alternative view is that the Antarctic ice 214 147 sheet had stabilized by 14 Ma [35], implying that the majority of 215 148 glacial erosion had already occurred by this time [64] and that the 216 149 bedrock topography may have been similar to modern after the 217 150 Fig. 1. Schematic of model coupling procedure, showing variables passed middle Miocene. Experiments were performed for two bedrock 218 151 between models and timestep of couplings. topography scenarios, one is the modern bedrock topography 219 152 (Scenario A) and the second is an approximation of the Miocene 220 153 allowing grounding lines to advance seaward to the continental bedrock topography (Scenario B).
Details
-
File Typepdf
-
Upload Time-
-
Content LanguagesEnglish
-
Upload UserAnonymous/Not logged-in
-
File Pages7 Page
-
File Size-