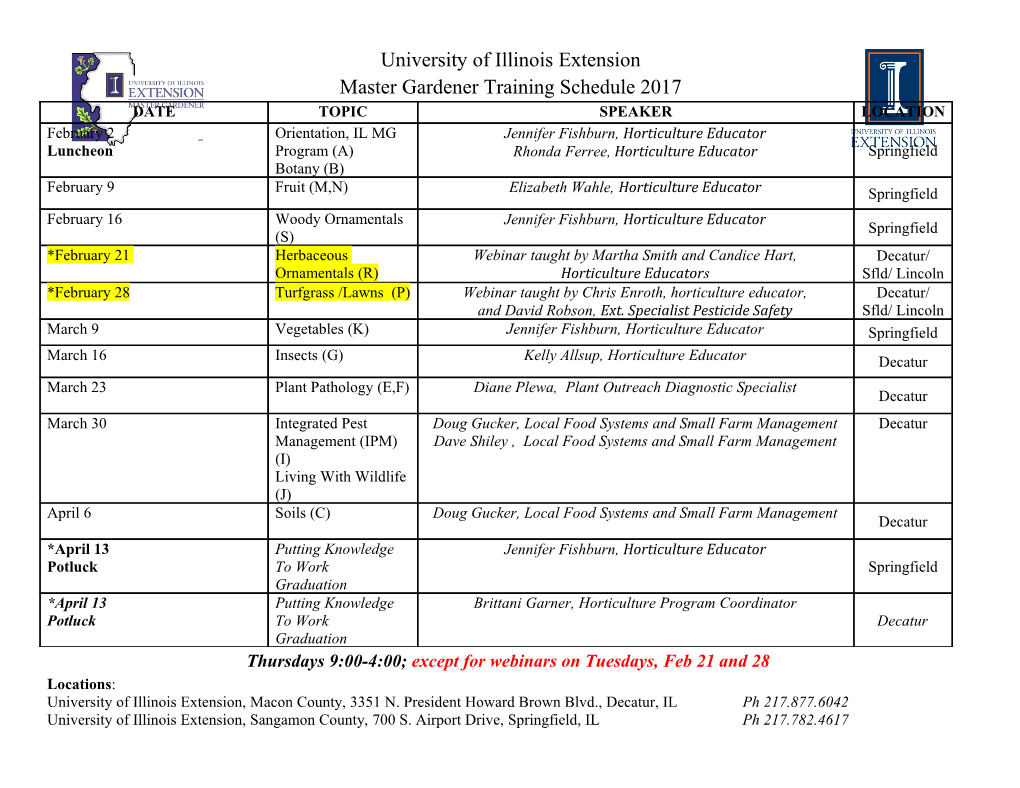
ARTICLE IN PRESS Quaternary Science Reviews 26 (2007) 37–55 Glacial variability over the last two million years: an extended depth-derived agemodel, continuous obliquity pacing, and the Pleistocene progression Peter Huybersà Department of Geology and Geophysics, Woods Hole Oceanographic Institution, Woods Hole, MA, 02540, USA Received 11 November 2005; received in revised form 21 May 2006; accepted 21 July 2006 Abstract An agemodel not relying upon orbital assumptions is estimated over the last 2 Ma using depth in marine sediment cores as a proxy for time. Agemodel uncertainty averages Æ10 Ka in the early Pleistocene (2–1 Ma) and Æ7 Ka in the late Pleistocene (1Ma to the present). Twelve benthic and five planktic d18 O records are pinned to the agemodel and averaged together to provide a record of glacial variability. Major deglaciation features are identified over the last 2 Ma and a remarkable 33 out of 36 occur when Earth’s obliquity is anomalously large. During the early Pleistocene deglaciations occur nearly every obliquity cycle giving a 40 Ka timescale, while late Pleistocene deglaciations more often skip one or two obliquity beats, corresponding to 80 or 120 Ka glacial cycles which, on average, give the 100 Ka variability. This continuous obliquity pacing indicates that the glacial theory can be simplified. An explanation for the 100 Ka glacial cycles only requires a change in the likelihood of skipping an obliquity cycle, rather than new sources of long-period variability. Furthermore, changes in glacial variability are not marked by any single transition so much as they exhibit a steady progression over the entire Pleistocene. The mean, variance, skewness, and timescale associated with the glacial cycles all exhibit an approximately linear trend over the last 2 Ma. A simple model having an obliquity modulated threshold and only three adjustable parameters is shown to reproduce the trends, timing, and spectral evolution associated with the Pleistocene glacial variability. r 2006 Elsevier Ltd. All rights reserved. Keywords: Glacial cycles; Mid-Pleistocene transition; Geochronology; Obliquity; Orbital forcing; Hypothesis test 1. Introduction contrast, late-Pleistocene (1 Ma-present) glacial cycles have a longer 100 Ka timescale often attributed to orbital The onset of glaciation near 3 Ma is thought to owe to precession (Hays et al., 1976; Imbrie et al., 1992; Ghil, a gradual cooling trend over the last 4 Ma (Shackleton 1994). and Hall, 1984; Raymo, 1994; Ravelo et al., 2004) which is Existing hypotheses for this ‘‘mid-Pleistocene transition’’ itself part of a longer-term trend over the last 50 Ma (or mid-Pleistocene revolution) from 40 to 100 Ka glacial (Zachos et al., 2001). Early-Pleistocene (2–1 Ma) glacial cycles call for shifts in the controls on glaciation to activate cycles have a 40 Ka timescale; thus these cycles are new sources of low-frequency variability (Saltzman and readily attributed to the 40 Ka changes in Earth’s obliquity Sutera, 1987; Maasch and Saltzman, 1990; Ghil, 1994; (e.g. Raymo and Nisancioglu, 2003; Huybers, 2006). In Raymo, 1997; Paillard, 1998; Clark et al., 1999; Tziperman and Gildor, 2003; Ashkenazy and Tziperman, 2004). The recent results of Huybers and Wunsch (2005) (hereafter à Harvard University, Earth and Planetary Sciences, Museum Bldg. HW05), however, show that the late Pleistocene glacial rm405, 20 Oxford St., Cambridge MA 02138, USA. Tel.: +1 617 233 3295; fax: +1 508 457 2187. terminations are paced by changes in Earth’s obliquity, E-mail addresses: [email protected], [email protected]. suggesting that a more unified glacial theory is possible, 0277-3791/$ - see front matter r 2006 Elsevier Ltd. All rights reserved. doi:10.1016/j.quascirev.2006.07.013 ARTICLE IN PRESS 38 P. Huybers / Quaternary Science Reviews 26 (2007) 37–55 related to obliquity both during the early and late 2.1. Converting depth to age Pleistocene. Here, the argument is made that the progres- sion from 40 to 100 Ka glacial cycles is not marked by Conversion of sediment core depths into age estimates is any specific transition and does not require any real change based on the graphic correlation methodology of Shaw in the physics of glacial cycles. (1964) and detailed in HW04. Only those instances where The paper is organized as follows: In Section 2 the depth- the methodology is extended or altered from HW04 are derived agemodel of Huybers and Wunsch (2004) (here- dwelled on here. Geomagnetic age control comes from the after HW04) is extended from 0.8 to 2 Ma. The reader not Brunhes–Matuyama transition (B–M) (0.78 Ma), the interested in agemodel construction may safely skip this Jaramillo (0.99–1.07 Ma), and Olduvai (1.77–1.95 Ma) section. In Section 3 the hypothesis testing procedures subchrons, and the Matuyama–Gauss transition outlined in HW05 (but now applied to many more (2.58 Ma) (Berggren et al., 1995; Cande and Kent, 1995). observations) are used to evaluate the relationship between These geomagnetic ages are derived from a combination of orbital variations and glacial variability. Section 4 discusses radiometric dating techniques, sea-floor spreading rates, the trends in Pleistocene glacial variability, and Section 5 and astronomically derived age estimates—the last indicat- presents a simple model which describes these trends and ing that geomagnetic age estimates do contain limited the timing of the glacial cycles. The paper is concluded in orbital assumptions. However, only six geomagnetic ages Section 6. are utilized in the course of 2 Ma and the orbital assumptions are but one constraint on the geomagnetic ages, minimizing the influence of the orbital assumptions. Also, the B–M is radiometrically dated to sufficient 2. The timing of Pleistocene glaciation accuracy ð2KaÞ (Singer and Pringle, 1996) that it is essentially independent of orbital assumptions, and thus so The use of age estimates not depending on orbital is the agemodel between the present and 0.78 Ma. assumptions are required to avoid circular reasoning when Geomagnetic events are assumed to occur in the same assessing the link between glacial and orbital variability. isotopic stages identified by Raymo and Nisancioglu (2003) This section presents an extension of the depth-derived for core DSDP607. agemodel of HW04 from 0.8 to 2.0 Ma. This agemodel References and statistics for each sediment core used in improves on previous non-orbitally tuned Pleistocene age this study are given in Table 1. Approximately synchro- estimates (Williams et al., 1988; Raymo and Nisancioglu, nous time horizons are identified between sediment cores 2003) by combining numerous age–depth relationships, by identifying corresponding d18 O events in the separate correcting for down-core compaction, and by providing for stratigraphies. (Event synchronization is limited by the age uncertainty estimates. Most agemodels spanning the signal propagation time through the ocean and the Pleistocene (e.g. Shackleton et al., 1990; Lisiecki and resolution of the d18 O stratigraphy.) HW04 identified 17 Raymo, 2005) constrain ages by aligning variations in the events over 780 Ka, giving an average event spacing of d18 O record with variations in the orbital parameters, thus 46 Ka. Here, a total of 104 d18 O events are identified precluding an objective evaluation of the orbital influence between the present and the Matuyama–Gauss transition on glacial timing. providing an average d18 O event spacing of 24 Ka (see Table 1 Sediment cores Name Reference Species S¯ nt Lat. Lon. DSDP607 Ruddiman et al. (1989) B 4.0 3.5 41 N 33 W MD900963 Bassinot et al. (1994) P 4.6 2.3 5 N 74 E ODP663 de Menocal et al. (unpublished) P 3.9 3.0 1S 12 W ODP664 Raymo (1997) B 3.7 3.4 0 23 W ODP677 Shackleton et al. (1990) B,P 3.9 2.1,1.8 1N 84W ODP846 Mix et al. (1995a) B 3.7 2.5 3S 91 W ODP849 Mix et al. (1995b) B 2.9 3.6 0 111 W ODP925 Bickert et al. (1997) and Curry and Cullen (1997) B 3.7 2.2 4 N 43 W ODP927 Cullen and Curry (1997), and Curry and Cullen (1997) B,P 4.5 3.2,2.2 6 N 43 W ODP980 Flower (1999), McManus et al. (1999, 2002), and Oppo et al. (1998, 2001). B 12.3 1.6 55 N 17 W ODP982 Venz et al. (1999). B,P 2.5 2.3,2.0 57 N 18 W ODP983 Channell et al. (1997) and McManus et al. (2003) B 11.4 0.9 61 N 22 W TT013-PC18 Murray et al. (2000) B 1.5 3.7 2S 140 W TT013-PC72 Murray et al. (2000) B 1.6 3.3 0 139 W Characteristics and primary references for each core. Columns from left to right display d18 O species benthic (B) and/or planktic (P), the mean sediment accumulation rate (S¯ , cm/Ka), the mean interval between d18 O measurements ðnt; KaÞ, and the latitude and longitude of each core site. ARTICLE IN PRESS P. Huybers / Quaternary Science Reviews 26 (2007) 37–55 39 time (Ky) 2000 1800 1600 1400 1200 1000 800 600 400 200 0 10 9 odp663 8 7 odp927 6 odp982 5 O anomalies 4 18 md900963 δ 3 2 odp677 1 1 11.3 17.1 9.1 5.3 31 25 15.3 7.3 53 37 21 19.1 15.1 7.1 69 63 55 49 47 45 33 29 27 13.1 13.1 5.1 71 67 65 61 57 43 35 11.1 8.5 O 51 6 56 41 39 18.3 14 0 73 70 59 28 12 10 8 18 46 44 23 18 16 14.3 68 54 50 30 26 16.4 3.3 2 δ 72 66 62 60 48 42 32 12.3 8.3 6.5 52 36 24 64 58 40 34 16.3 38 20 1 22 2000 1800 1600 1400 1200 1000 800 600 400 200 0 time (Ky) Fig.
Details
-
File Typepdf
-
Upload Time-
-
Content LanguagesEnglish
-
Upload UserAnonymous/Not logged-in
-
File Pages19 Page
-
File Size-