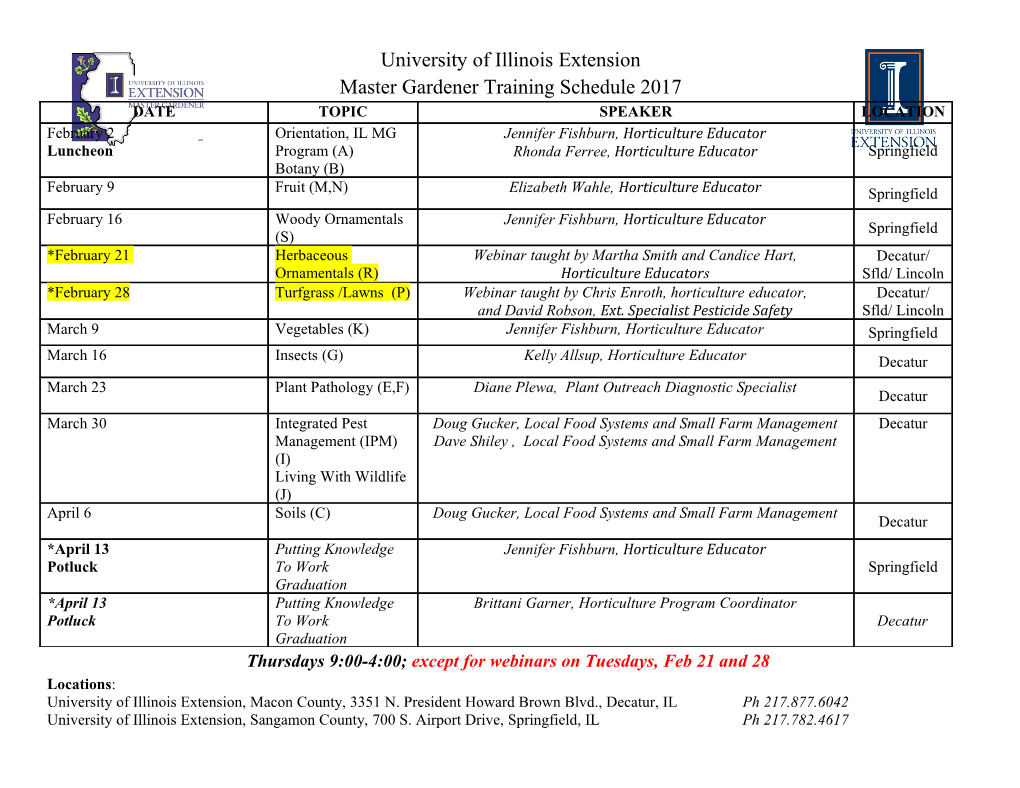
VOLUME 138 MONTHLY WEATHER REVIEW JANUARY 2010 A Diagnostic Study of the Intensity of Three Tropical Cyclones in the Australian Region. Part I: A Synopsis of Observed Features of Tropical Cyclone Kathy (1984) FRANCE LAJOIE AND KEVIN WALSH School of Earth Sciences, University of Melbourne, Parkville, Australia (Manuscript received 20 November 2008, in final form 13 May 2009) ABSTRACT Objective streamline analyses and digitized high-resolution IR satellite cloud data have been used to ex- amine in detail the changes in the environmental circulation and in the cloud structure that took place in and around Tropical Cyclone Kathy (1984) when it started to intensify, and during its intensification and dissi- pation stages. The change of low-level circulation around Tropical Cyclone Kathy was measured by the change in the angle of inflow (a4) at a radius of 48 latitude from the cyclone center. When Kathy started to intensify, a4 increased suddenly from 208 to 42.58 in the northerly airstream to the north and northeast of the depression, and decreased to 08 to the south of the depression. At that stage the low-level circulation around the depression appeared as a giant swirl that started some 600 km to the north and northeast of the depression and spiraled inward toward its center, while trade air, which is usually cool, dry, and stable, did not enter the cyclonic circulation. The angle a4 remained the same during intensification. During the dissipation stage, a4 returned to 208 and trade air started to participate in the cyclonic circulation. Satellite cloud data were used to determine the origin, evolution, and importance of the feeder bands in the intensification of the cyclone, to follow the moist near-equatorial air that flowed through them and to estimate the maximum height of cumulonimbi that developed in them, to observe the changes in the convective activity in the central dense overcast (CDO) area, as well as in the area around the CDO. Most of the observed changes in Kathy have also been observed in other tropical cyclones during intensification and dissipation. Using the sequence of observed changes of the circulation and of convective activity in and around the CDO of Kathy, a mathematical model has been developed to forecast the intensity of a tropical cyclone. The model and its application to three tropical cyclones in the Australian region are described in Part II of this paper. 1. Introduction a tropical cyclone without having to consider these elusive parameters and processes. Meteorological parameters and physical atmospheric processes that can influence the intensification of a a. Tropical cyclone development processes tropical cyclone have been identified and discussed by Processes that have been shown to influence tropical many investigators. Apart from having insufficient con- cyclone development are as follows. ventional observations to accurately determine the mag- nitude of these parameters or processes, none of the latter 1) THE SEA SURFACE TEMPERATURE is consistent all the time as any of them can produce When considering a large number of tropical cyclones, intensification or weakening in one cyclone but not nec- the minimum central surface pressure p is found to be essarily in others. We shall briefly consider this problem c related to the SST between 268 and 308C: the greater the below. In Lajoie and Walsh (2010, hereafter Part II), we SST the lesser is p (Miller 1958; Titley and Elsberry will discuss a model that can forecast, with a lead time of c 2000). This relationship appears to break down for 15 (possibly 30) h, the central surface pressure, as well as SST . 308C (Merrill 1988; Evans 1993; DeMaria and the radial and the azimuthal wind distribution around Kaplan 1994), with only a few intense storms being ob- served at these temperatures (Kaplan and DeMaria 2003). 2) RELATIVE HUMIDITY Corresponding author address: Kevin Walsh, School of Earth Sciences, University of Melbourne, Parkville VIC, 3010 Australia. High relative humidity (.70%) either in the boundary E-mail: [email protected] layer (Schade and Emanuel 1999) or in the 850–700-hPa DOI: 10.1175/2009MWR2875.1 Ó 2010 American Meteorological Society 3 Unauthenticated | Downloaded 10/01/21 03:15 PM UTC 4 MONTHLY WEATHER REVIEW VOLUME 138 layer (Kaplan and DeMaria 2003) plays a significant role flow by an axisymmetrization process that causes the in the development and intensification rate of a tropical tangential winds at a radius outside the initial RMW to cyclone. The latter authors, however, found that only increase, thus causing the vortex to intensify and the about 66% (80%) of tropical cyclones with relative hu- RMW to expand. This process can also produce a sig- midity greater than 70% (.80%) in the 850–700-hPa nificant increase of intensification rate (Montgomery layer undergo rapid intensification, defined as either and Enagonio 1998; Moller and Montgomery 2000). On an increase of sustained mean surface wind of at least the other hand, Bister (2001) has argued that the latent 15.4 m s21 (30 kt) in 24 h or a decrease of central sur- heat released in the rainbands of the VRWs reduces the face pressure of at least 15 hPa in 24 h. radial pressure gradient and causes the tropical cyclone to weaken. 3) VERTICAL WIND SHEAR 6) LOW-LEVEL INWARD FLUX OF MOMENTUM In general, if the vertical shear of environmental horizontal winds between 850 and 200 hPa is less than Low-level environmental forcing can also produce 5ms21, a tropical cyclone may intensify, but no in- intensification of a tropical cyclone. Using conventional tensification is likely to occur if the shear is 10–15 m s21 surface wind fields, Molinari and Skubis (1985) dis- (Simpson and Riehl 1958; Gray 1979; McBride and Zehr cussed a case of wavelike perturbation, characterized by 1981; Merrill 1988; Zehr 1992; DeMaria and Kaplan a surge of inflow and upward vertical motion, that de- 1994; DeMaria 1996; Hanley et al. 2001; Kimball and veloped at a radius of 1600 km on the equatorward and Evans 2002; Kaplan and DeMaria 2003). A few tropical east side of a tropical depression, and that propagated cyclones have however been observed to intensify in inward toward the depression at 15 m s21 to reach the face of strong vertical shear (Molinari 1998). storm center 36 h after its development. Molinari and Skubis used a momentum budget to show that the in- 4) UPPER-TROPOSPHERE TROUGH INTERACTION ward flux of momentum contributed to a significant The interaction between an upper-air trough and momentum source within 440 km of the center of the a tropical cyclone can also play an important role in its depression and produced a rapid intensification of the intensification (Molinari et al. 1995, 1998; Emanuel depression when the surge reached its center. 1997; Shi et al. 1997) and in its rapid intensification 7) OTHER ATMOSPHERIC PROCESSES (Titley and Elsberry 2000; Kaplan and DeMaria 2003). Yet, using a 3-yr data sample, Hanley et al. (2001) found Vortex Rossby wave (VRW) rainbands associated that only 61% of all distant large-scale troughs and 78% with extensive cirrostratus and convective clouds (May of all small-scale troughs caused storms to intensify, 1996) do sometimes extend to 150 km from the cyclone while in 82% of no-trough cases the tropical cyclone also center (Kuo et al. 1999; Chow et al. 2002; Wang 2002). intensified. Hanley et al. (2001) also found that 30% of The vertical gradient of diabatic heating that results no-trough cases exhibit rapid intensification ($20 hPa from the release of latent heat in the stratiform cloud in 12 h), compared to 16% for small-scale troughs. and the evaporational cooling below the cloud base produces a PV concentration in the midtroposphere 5) VORTEX ROSSBY WAVES (Raymond and Jiang 1990). This PV concentration is Vortex Rossby waves can develop on both sides of the sometimes associated with midlevel jets (May 1996). radius of maximum wind (RMW) of a tropical cyclone While May and Holland (1999) have argued that this PV because of the large potential vorticity (PV) gradients production can be transported into the inner core and in these areas (Montgomery and Kallenbach 1997; Kuo causes intensification, Bister (2001) has suggested that et al. 1999; Corbosiero et al. 2006). When they evolve the latent heat released by the rainbands reduces the within the RMW, their associated convective clouds give radial pressure gradient, which then weakens the tropi- rise to asymmetries in the eyewall (Kuo et al. 1999; cal cyclone. Kossin and Schubert 2002; Chen and Yau 2001; Wang The PV concentration in the midtroposphere can also 2001, 2002), but when they evolve outside the RMW, initiate mesoscale vortices that are associated with outer they manifest themselves as spiral rainbands that de- rainbands beneath the outflowing cirrostratus bands velop within 50–80 km from the cyclone center and that (Houze 1977; Zipser 1977). Whether this process plays move cyclonically around the vortex while traveling a positive or negative role in the intensification of a trop- outward from the RMW (Kimball and Evans 2002). ical cyclone is not known yet (Zhang et al. 2002). According to Montgomery and Kallenbach (1997) and The large number of environmental parameters and Moller and Montgomery (1999), the energy of the atmospheric processes that can affect the intensity of VRWs is transferred from the asymmetry into a circular a tropical cyclone one way or another makes intensity Unauthenticated | Downloaded 10/01/21 03:15 PM UTC JANUARY 2010 L A J O I E A N D W A L S H 5 forecasting one of the most challenging tasks of the meteorologist. Let us now consider some of the tech- niques that are used to predict the intensity of tropical cyclones and their general performance.
Details
-
File Typepdf
-
Upload Time-
-
Content LanguagesEnglish
-
Upload UserAnonymous/Not logged-in
-
File Pages19 Page
-
File Size-