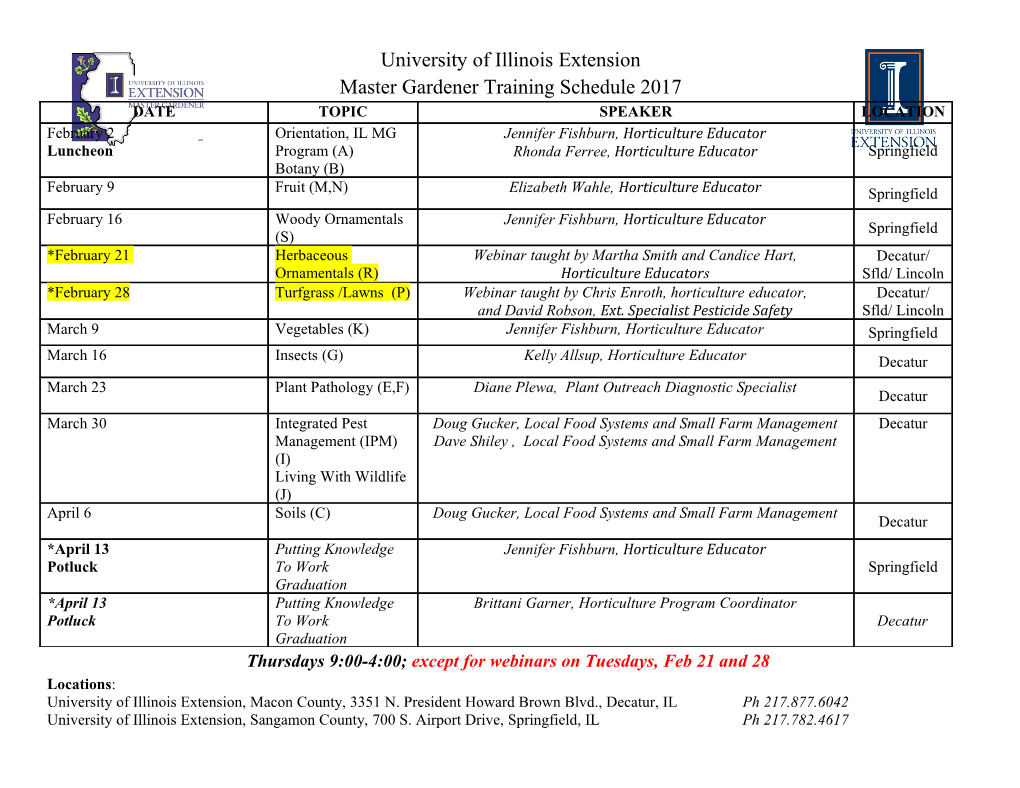
ADOBE CORPORATE CAMPUS UTAH, USA – STRUCTURE BECOMES THE ARCHITECTURE GEOFF S SHARP Senior Project Engineer, Holmes Consulting Group LP SUMMARY Phase one of Adobe Corporation’s new corporate campus is an example of where the thoughtful selection of structural system and construction materials allowed for seamless integration of engineering and architecture. Constructed of post tensioned and conventionally reinforced concrete the office structure showcases what can be achieved when the structure becomes the architecture. At over 200 metres in length and boasting cantilevered frames and a 25 metre “bridge” the use of concrete was essential to the success of this new office building. INTRODUCTION In late 2012, United States based software giant Adobe Corporation moved into their new 28,000 square metre campus in Lehi, Utah. The decision to expand away from their long standing Silicon Valley home required their new facility to make a statement. It needed to attract a new class of outdoors-loving young professionals. Located approximately 50km south of Salt Lake City, the resulting buildings form stage one of their impressive new home on the “Silicon Slopes” of Utah. Challenges included a highly sloped site with significant variation in subsoil conditions and a dual carriage roadway dividing the property. Figure 1 below shows a rendering of the phase one buildings Figure 1 - Phase 1 Adobe Campus Rendering, courtesy of WRNS Studio The lead architects for the project were WRNS Studio of San Francisco with structural engineering delivered through a collaboration between Holmes Culley in San Francisco and Dunn Associates in Salt Lake City. The design process between architect and engineer was very successful due to an open and collaborative approach. The new campus consists of a four storey office structure, three level gymnasium and café, with an interconnecting atrium. The reinforced concrete office structure is over 200 metres in length and 24 metres in width. The structural steel gymnasium and atrium are defined by the 35 metre clear span roof which frames a 14 metre high glazed wall providing fantastic views of the Wasatch Mountains. The focus of this paper is the cast insitu concrete office building. THE OFFICE BUILDING From an architectural standpoint the office building needed to be a very functional working space for the thousands of employees who now work there, while making a statement aesthetically. On the engineering front, significant effort was spent optimising the structural grid to best suit the workspace layouts. This involved an optioneering exercise evaluating possible floor systems and their potential spans. In addition to the usual challenges associated with maximising usable floor area, the building was required to cantilever over eight metres at each end and “bridge” a 25 metre roadway. To split the building for seismic and thermal reasons a movement joint was introduced adjacent to the main entrance. Figure 2 below shows a typical office building floor plan with the cantilevered ends of the building and plan location of the bridge shaded. Figure 2 - Typical office building floor plan General Structural System After a detailed evaluation of various construction materials and approaches the decision was made to adopt reinforced concrete and leave it predominantly exposed. This saved money on finishes and accentuated the audacity of the architecture. Given the desire for open floor plates with limited visual breaks, a long-span floor system was required. The chosen system was a one-way spanning 200mm thick post tensioned flat slab. This allowed the transverse cross section of the typical floor plate to have long internal spans of seven and eleven metres with three metre cantilevers overhanging at each edge. In the longitudinal direction, reinforced concrete beams span nine metres. In order to verify the suitability of these relatively long spans, finite element analyses were undertaken to ensure that excessive floor vibration and long term creep deflections would not undermine performance. The adjacent long spans and cantilevered edges provided load balancing efficiency due to the concept of continuity, something which is difficult to achieve with the precast floors typically used in New Zealand. The level of finish achieved at the floor soffit with flat slab construction became particularly important architecturally as there are very few ceilings. Figure 3 - Finite Element Modelling of Flat Slab Floors - ADAPT Located in Utah, the lateral design of the building was governed by seismic loads. The longitudinal lateral bracing system consists of reinforced concrete moment resisting frames, with shear walls in the transverse direction. Both were detailed to the “special” requirements of American Concrete Institute, ACI-318, due to the high seismicity of the region. In order to accommodate the atypical bridge and cantilever areas of the building, the lateral bracing systems had to be detailed accordingly. For the cantilevers a “pin” detail was introduced to prevent the gravity structure from contributing to the lateral stiffness of the building. In addition the shear walls needed to be separated from the post tensioned floors during construction. This was to prevent the pre-stress force unintentionally loading the shear walls during stressing of the floors. Practically this was achieved by leaving localised pockets of conventionally reinforced concrete slab each side of the shear walls which were not poured until after the floors around them had been stressed. Figure 4 below consists of two photographs taken during construction of these delayed pour strips. Figure 4 Delayed pour strips were left in the post tensioned slabs until after the slabs were stressed. Adding a glazed curtain wall cladding system to the architecturally exposed structure emphasized a sense of regularity to the upper floors. By comparison, a deliberate “grounding” effect was sought for the lower floors of the office building. These floors accommodate around nine metres of variation in existing ground elevation. To effectively anchor the building both visually and structurally a series of exposed concrete shear walls were introduced in both directions. The heft and seemingly random height of these walls develops a contrast with the rhythm and elegance of the glass box above. From a functional standpoint these anchor points form the foundation levels from which the moment frames and upper level shear walls rise. Figure 5 below shows the glass box of the upper floors above the “grounding” lower floor shear walls. Figure 5 - The Eastern End of the Office Building – Photo credit, Blake Marvin With the architecture established the main structural engineering challenge was to bridge the roadway and cantilever the ends of the building without upsetting the abovementioned sense of rhythm. It was important that someone on the inside of the building could move through the typical floor plate and over the bridge or cantilevers without a discernable change in the structural system. The Bridge The 15 acre site purchased by Adobe for their new campus is split into two main parcels by an existing four lane public road. To connect the site the phase 1 office building needed to be able to span 25 metres without a discernable change in the structural system. The position of this roadway is indicated in Figure 6 below. Figure 6 - Adobe Way cuts through the middle of the site The structural solution for the bridge was a mixture of Vierendeel truss and concrete shear panel elements. The upper three levels of the building above the bridge consist of the typical moment resisting frame, which due to its lateral bracing function has sufficient stiffness to contribute to spanning the roadway. The bulk of the work however, is done by the lowest floor of the bridge where two of the panels between the beams and columns are in-filled with 450mm thick reinforced concrete. These shear panels work in conjunction with the beams above and below which complete the load path. A compression strut forms in the beam above the shear panels and uses the typical frame beam. Below the panels a concrete encased structural steel element was anchored each side of the road to provide tension continuity. Figure 7 below shows graphically how the bridge portion resists vertical loads. Figure 7 - Cross Section through the bridge showing main structural components Under lateral loads the bridge panels had to be less stiff than the adjacent “abutment” portions of the building to prevent the potential for overloading during an earthquake. In order to verify that this bridge component of the building was compatible with the remainder of the lateral bracing system a 3D analysis model of the building was developed. A great deal of care was required in the detailing of this portion of the project. Figure 8 - Temporary shoring in place as the bridge portion of the building is constructed Making this element of the building particularly complicated was the need to construct over an active roadway. As such the lower portion of the bridge was also designed for a number of construction stage load cases. This allowed the shear panels and tension / compression elements to be built first, along with the first two slabs, to create the platform on which the remainder of the building could be constructed. Taking this approach limited road closures to half of roadway at any one time. Figure 8 above shows the construction of the bridge with the open roadway while Figure 9 below shows the finished building. Figure 9 - The completed bridge portion of the office building – Photo credit, Blake Marvin The Cantilevers As described previously, one of the architectural aims was to accentuate the regular, linear nature of the typical office floors which float above the heavier anchor points. Key to the success of this was having the ends of the office building extend beyond the founding level below. Each end of the office building cantilevers one bay of over eight metres.
Details
-
File Typepdf
-
Upload Time-
-
Content LanguagesEnglish
-
Upload UserAnonymous/Not logged-in
-
File Pages8 Page
-
File Size-