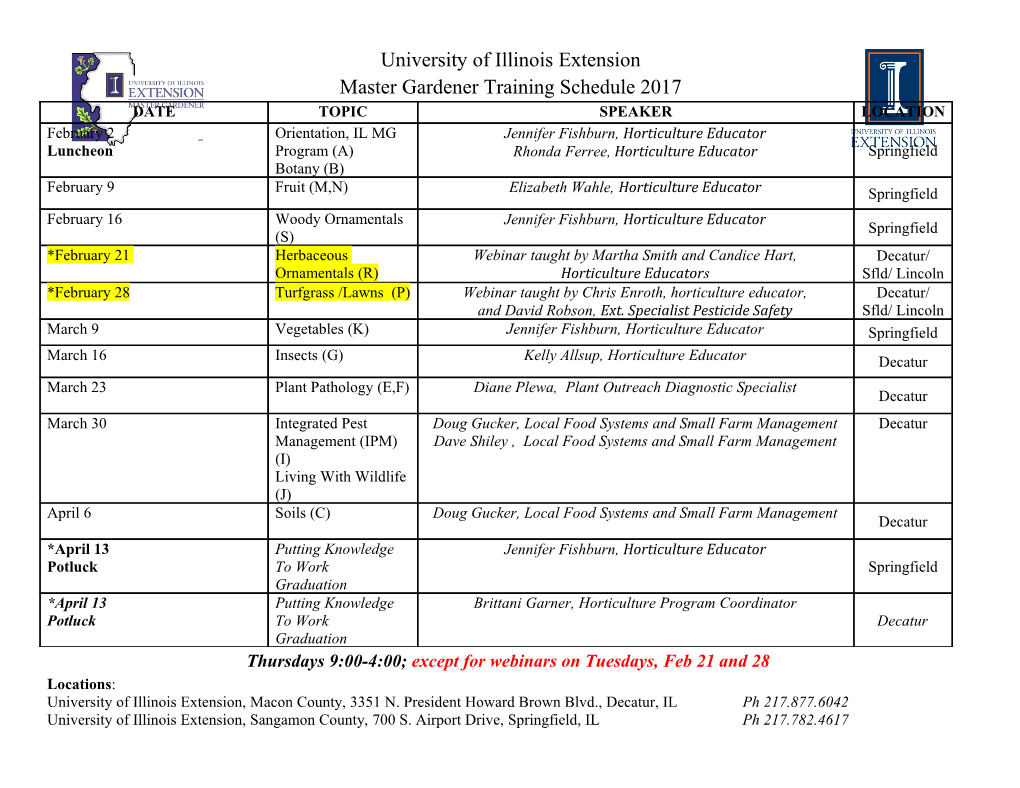
UNIVERSITY OF CINCINNATI Date: 1-Oct-2009 I, Evan T Ogburn , hereby submit this original work as part of the requirements for the degree of: Master of Science in Chemistry It is entitled: Characterization of a Microfabricated Electrochemical Detector and Coupling with High Performance Liquid Chromatography Student Signature: Evan T Ogburn This work and its defense approved by: Committee Chair: William Heineman, PhD William Heineman, PhD Carl Seliskar, PhD Carl Seliskar, PhD 11/12/2009 288 Characterization of a Microfabricated Electrochemical Detector and Coupling with High Performance Liquid Chromatography A thesis submitted to the Division of Research & Advanced Studies of the University of Cincinnati In partial fulfillment of the requirements for the degree of Master of Science In the Department of Chemistry of the College of Arts and Sciences 2009 By Evan Ogburn B.A., Earlham College, 2005 Committee Chair: William R. Heineman, Ph.D. Abstract A disposable micro-fabricated electrochemical cell has been developed, characterized with multiple electrochemical systems, and coupled with high performance liquid chromatography to form a high performance liquid chromatography electrochemical detection (HPLC-ED) system. The detection system consisted of the micro-fabricated electrochemical detector, a flow-cell and a fixture mounted with electrical connections leading from the detector to the potentiostat. The detector is easy to fabricate, inexpensive, and maintains a high performance level which makes it a practical choice for electrochemical detection. The simplicity of the fabrication process for this detector allows it to be used as a disposable device that can be replaced easily if its performance degrades. Parameters for the optimization of the performance were studied in a three-electrode system with a special focus on HPLC-ED, using ascorbic acid, acetaminophen, and potassium ferricyanide as model compounds. A separation of three pharmaceutical compounds, dextrorphan, levallorphan, and acetaminophen was also performed in order to demonstrate the performance of the novel detector in comparison to commercially available macro electrochemical detectors. It was determined that the micro-fabricated detector’s performance was comparable to traditional non-disposable electrodes in these systems, especially HPLC-ED, which was the system of focus. The calibration curves constructed for each model compound showed linearity (R2> 0.99), the limit of detection reached picomolar (< 100) levels, and the peaks generated were more resolved and free from interference when the micro-fabricated detector was used. When this novel detector was used, the limit of detection reached 1.00 nM for acetaminophen, 1.00 nM for ascorbic acid, 50.0 nM for dextrorphan and 80.0 nM for levallorphan. When the nondisposable commercially available detector was used, the limit of detection reached 0.0500 nM for acetaminophen and 1.00 nM for ascorbic acid. Dextrorphan iii and levallorphan were detected as low as 2.5 nM and 5.00 nM, respectively, with the commercial system when samples were diluted in mobile phase, but the signal reproducibility was not good when tested in human plasma. The results within this thesis demonstrate that a micro-fabricated electrochemical detector can operate at a high level of performance when used in HPLC-ED, while maintaining cost effectiveness. iv v Acknowledgements I would like to convey my genuine pleasure and gratitude to my advisor Dr. William R. Heineman from the Department of Chemistry, University of Cincinnati for giving me the opportunity to further my education and experience in the field of chemistry. My thanks are also extended to Dr. Carl J. Seliskar who has served on my committee from the beginning of my studies here at UC and Dr. Michael Dzwietakowski from Yellow Springs Instruments, Inc. for offering a great deal of kind help and knowledge throughout my studies. I would also like to thank all of the members of the Center for Chemical Sensors and Biosensors at UC as well as the members of Dr. Heineman’s and Dr. Seliskar’s group for support and guidance. I would like to acknowledge Yellow Springs Instruments, Inc. and the Indiana University School of Medicine Department of Clinical Pharmacology for providing the detectors and apparatus necessary to complete my research. Finally, I would like to thank my girlfriend, family and friends for the love and support they have shown me throughout the duration of my academic career. vi Table of Contents Abstract iii-iv Acknowledgements vi I. Introduction 1-3 Electrochemical Detection as It Occurs in HPLC-ED 3-5 The Basics of Chromatography and Its Use in HPLC-ED 5-7 Characteristics of HPLC-ED Separation and Detection 8-9 Development of a Novel HPLC-ED System 9-10 Background on Microfabricated Electrochemical Detectors Used in HPLC-ED 11-12 Electrochemical Detector Origin 12 Electrochemical Detector Description 12-16 Flow Injection Analysis (FIA) 17-18 Electrochemical Detection as It Occurs in FIA 18-19 Cyclic Voltammetry and How It Is Applied with Microfabricated Electrochemical Detectors 19-22 II. Experimental Chemicals 22 Standard Samples 23 Plating the Reference Electrode Within This System 23-27 HPLC-ED (Commercial System) 28 HPLC-ED (Novel System) 28-29 Flow Injection Analysis (FIA-ED) 29 Cyclic Voltammetry 30 III. Results Cyclic Voltammetry 30-33 Electrochemistry of Ascorbic Acid w/ Conventional and Microfabricated Electrodes 33-34 Flow Injection Analysis 34-38 HPLC-ED 38-40 Degradation of Detector and Signal 41-43 Analyzing Performance when the Degradation of a Novel Microfabricated Electrode Begins 43-44 The Effect of Concentration of Analyte on Electrochemical Detection in HPLC-ED 44-46 Comparing the Novel HPLC-ED System with a Commercialized System 46-47 Limit of Detection 48-50 Band Broadening as a Result of Longitudinal Diffusion 50-54 Chromatographic Properties of Each System 54-56 Separation of Pharmaceutical Compounds in Multiple Matrices 56-58 Chromatographic Attributes 59-67 IV. Conclusion 67-68 References 69 vii List of Figures and Tables Figure 1. L-ascorbic acid (A) and acetaminophen (B) chemical structures. Both compounds were used as model analytes to test the performance of the electrochemical detector. 10 Figure 2. Schematic of the microfabricated electrochemical detector presented within this thesis. 13 Figure 3. (A) A photograph of the entire electrochemical detector presented in this thesis. The circles located on the detector are valve connections allowing liquid to enter and leave the flowcell apparatus. (B) Close up photograph of the electrodes on the detector. The dimensions of these electrodes are listed in Table 1. 15 Table 1. Dimensions of the microfabricated electrochemical detector and coupled microfluidic device. 15 Figure 4. Image of solution fluidic flow channel found in the flowcell used to couple the microfabricated electrochemical detector with FIA and HPLC. 16 Figure 5. A microfluidic-chip that is used to direct fluid flow across the surface of the electrodes on the microfabricated detectors, enabling electrochemical detection. 18 Figure 6. Potential vs. Time, excitation signal for cyclic voltammetry. 20 Figure 7. CV of 4 mM potassium ferricyanide in 1M KNO3 initiated at 0.8 V potential vs. Ag/AgCl in negative direction at 20 mV/s. Gold working electrode used, area = 2.01 mm2. 21 Figure 8. (A) Uniform layer of Ag/AgCl plated on gold to form the reference electrode and (B) a layer that is nonuniform. Environmental scanning-electron microscope (ESEM) images of a uniformly plated (C) and non-uniformly plated (D) reference electrode in which plating was performed simultaneously. 25 Figure 9. CV of 4 mM K3Fe(CN)6 in 1 M KNO3 initiated at 0.8 V in the negative direction at a scan rate of 20 mV/s using (A) the detector pictured in Fig. 8A, uniform plating and with (B) the detector in Fig. 8B, non-uniform plating. 31 Figure 10. (A) CV of a conventional size gold working electrode in a solution of 4 mM K3Fe(CN)6 in 1 M KNO3. (B) CV of the same solution obtained with the microfabricated electrochemical detector presented in this paper. 32 Figure 11. Effect of varying scan rate on cyclic voltammetry of 10 mM ascorbic acid in 10 mM KH2PO4 using microfabricated electrochemical detectors. 34 Figure 12. Electrochemistry, performed with the FIA-ED system, of 2 mM ferricyanide in buffer B at a potential of -75mV. The pressures at which the results were recorded are labeled appropriately. 35 Figure 13. Average peak current (µA) of 2mM potassium ferricyanide analyzed in the FIA-ED system at a working potential of -75 mV. The pressure applied to induce flow was varied between 0.5, 1.2, 0.7, 0.5 and 0.3 psi. The run time for this analysis was 48 h. 36 Figure 14. Peak current (µA) of 2mM potassium ferricyanide analyzed in the FIA-ED system at a working potential of -75 mV. The pressure applied to induce flow was 0.5 psi. The first 17 h of analysis is shown here. 37 Figure 15. Hydrodynamic voltammograms for acetaminophen (50 µM) (A) and ascorbic acid (100 µM) (B). 40 viii Figure 16. Stability of ascorbic acid (1 µM) and acetaminophen (0.25 µM) over a 10 hour period as shown by peak height. 41 Table 2. Stability of HPLC-ED Response of Acetaminophen (0.25 µM) and Ascorbic Acid (1.0 µM). 42 Figure 17. The response of acetaminophen (50 nM) using 3 different microfabricated electrochemical detectors of different condition, non-working (A), degrading (B) and new (C). 44 Figure 18. Calibration curve of ascorbic acid (A) and the effect of concentration on peak height (B) for acetaminophen detection by HPLC-ED using the microfabricated electrochemical detector. 45 Figure 19. HPLC-ED of acetaminophen (1 µM) examined with the novel microfabricated electrochemical detector (A) and a commercial detector (B). 46 Figure 20. Response for acetaminophen (1.0 x 10-9 M) using the novel HPLC-ED (A) and the response for acetaminophen (5.0 x 10-11 M) in a commerical HPLC-ED system (B).
Details
-
File Typepdf
-
Upload Time-
-
Content LanguagesEnglish
-
Upload UserAnonymous/Not logged-in
-
File Pages78 Page
-
File Size-