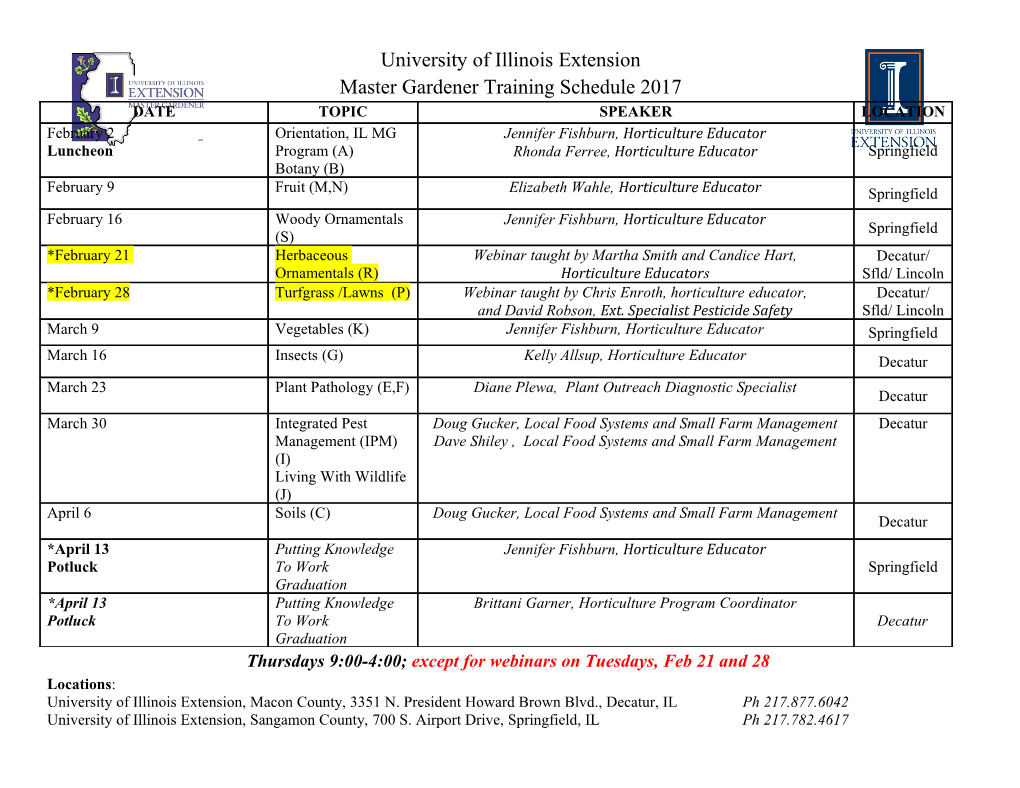
Scanning Microscopy Volume 6 Number 2 Article 2 4-6-1992 A Review of Graphite and Gold Surface Studies for Use as Substrates in Biological Scanning Tunneling Microscopy Studies Carol R. Clemmer University of Utah Thomas P. Beebe Jr. University of Utah Follow this and additional works at: https://digitalcommons.usu.edu/microscopy Part of the Biology Commons Recommended Citation Clemmer, Carol R. and Beebe, Thomas P. Jr. (1992) "A Review of Graphite and Gold Surface Studies for Use as Substrates in Biological Scanning Tunneling Microscopy Studies," Scanning Microscopy: Vol. 6 : No. 2 , Article 2. Available at: https://digitalcommons.usu.edu/microscopy/vol6/iss2/2 This Article is brought to you for free and open access by the Western Dairy Center at DigitalCommons@USU. It has been accepted for inclusion in Scanning Microscopy by an authorized administrator of DigitalCommons@USU. For more information, please contact [email protected]. Scanning Microscopy, Vol. 6, No. 2, 1992 (Pages 319-333) 0891- 7035/92$5.00+ .00 Scanning Microscopy International, Chicago (AMF O'Hare), IL 60666 USA A REVIEW OF GRAPHITE AND GOLD SURFACE STUDIES FOR USE AS SUBSTRATES IN BIOLOGICAL SCANNING TUNNELING MICROSCOPY STUDIES Carol R. Clemmer and Thomas P. Beebe, Jr.* Department of Chemistry and Center for Biopolymers at Interfaces, University of Utah, Salt Lake City, UT 84112 (Received for publication October 31, 1991, and in revised form April 06, 1992) Abstract Introduction The current status of biological Scanning Since Binnig and Rohrer first reported the Tunneling Microscopy (STM) investigations and the ability of the STM [8] to image deoxyribonucleic acid importance of using a well-characterized substrate (DNA) molecules [9], researchers have continued to are discussed. The findings of over two years of investigate the biological applications of the tech­ experiments and over 1,000 images obtained on gold nique. Researchers have been interested in improv­ substrates prepared by a variety of different methods ing the ability of STM to obtain structural informa­ are statistically summarized and compared to a very tion about biomolecules. In early 1989, the capability flat reference substrate, highly oriented pyrolytic of STM to resolve the major and minor grooves of un­ graphite (HOPG). In an effort to begin to corroborate coated DNA deposited onto HOPG was reported by STM results with those obtained from other more Beebe, et al. [6], and Lee, et al. [24]. Dunlap and established techniques, the results of Auger Electron Bustamante illustrated the possibility that STM Spectroscopy (AES) and Electron Spectroscopy for could be used to distinguish between the purine and Chemical Analysis (ESCA) of biomolecular STM pyrimidine bases of DNA in studies of uncoated samples are presented. poly(dA) molecules deposited onto HOPG and imaged in air [18]. Baldeschwieler and co-workers have pre­ sented results suggesting that STM can resolve DNA deposited onto HOPG and imaged in vacuum with atomic detail [17]. Although these results and others [5,6,9,17,18,23,24] have promoted the idea of STM as a technique useful for biological studies, they are not without problems. These problems involve the HOPG substrate upon which these studies were completed [13]. Although the majority of these ini­ tial biological STM experiments used HOPG as the substrate, a few researchers have completed their experiments on gold substrates [14,15,25]. A large body of the work completed on gold are in situ stud­ ies completed by S.M. Lindsay and co-workers. Now, seven years after the initial suggestion that STM could be applied to study biological sys­ tems, progress hinges critically on the need for better understanding of the technique and interpretation of the results. Several papers have discussed some of these ideas previously [7,10,13,33, and personal communications held at the Workshop on STM/AFM Key Words: scanning tunneling microscopy, highly and Molecular Biology held at Royaumont Abbey, oriented pyrolytic graphite, gold, Auger electron France, April 1991]. In this paper, the importance of spectroscopy, electron spectroscopy for chemical using a well-characterized, atomically flat substrate analysis, biomolecules, deoxyribonucleic acid is discussed. The results of comparative studies of HOPG (which was the substrate used for initial bio­ logical studies in this laboratory) and gold sub­ *Address for correspondence: strates, prepared by a variety of documented and Thomas P. Beebe, Jr. spoken methods are presented. University of Utah Further, the importance of verifying biological Department of Chemistry STM results with results collected from the more es­ Salt Lake City, UT 84112 tablished techniques of Scanning Electron Mi­ croscopy (SEM), Transmission Electron Microscopy Phone: (801) 581-5383 (TEM), AES, and ESCA is discussed. The results of FAX: (801) 581-8433 the analysis of STM samples by AES and ESCA illus- 319 C.R. Clemmer and T.P. Beebe, Jr. trate that depositions of biological molecules, specifi­ cally DNA, can be verified using these techniques. CoaxialKinetic Approach Scanning Tunneling Microscope Although it has been suggested that Scanning (cross-section view) Tunneling Spectroscopy (STS) could be used in prin­ coppergrounding shield ciple to differentiate a biomolecule from a substrate sampleholder wilh sample feature [1], this has not been demonstrated conclu­ sively in practice. Several researchers have verified their STM work using TEM and SEM [2- 4, 7 ,36,37,41]. ESCA has been used by researchers to verify surface modification of their substrate in conjunction with STM results [22,27,40]. STM has been shown to be a complimentary technique to TEM and SEM for the study of biological systems [4,36,41]. With improvements in experiment and theory, important biological questions can begin to quartz half-lube be addressed. ,___~5~c,,,.m _ __,.,I -STMbaseplate Experimental Methods ' FIGURE 1 - Schematic of the STM assembly in The STM used in this study consisted of a vertical cross-section. Schematic is drawn to scale. coaxial double-tube piezo design built at the Univer­ sity of Utah. The design has been described previ­ ously in detail [26,42]. Briefly, it consists of an outer piezoelectric tube responsible for sample x, y, z offset and macroscopic initial sample approach, and an dard surface analysis equipment (not including the inner piezoelectric tube responsible for the x, y, z STM) was obtained from Leybold-Heraeus as bolt-on scanning of the STM tip. The STM is shown components, which were attached to the custom de­ schematically in Figure 1. The tips were made of a signed UHV chamber. The UHV c~fmber has a base platinum/rhodium (H)l/o rhodium) alloy wire (0.51 pressure of less than 5.0 x 10- Torr which is mm diameter), and were prepared by two methods: achieved with a combination of ion, titanium subli­ ac etching [21] or mechanical cutting. All tips were mation, and turbomolecular pumps. A sample subjected to quality control experiments. Only tips transfer interlock facilitates the transfer of samples capable of obtaining atomic resolution in preliminary in and out of ultrahigh vacuum in approximately ten experiments on a freshly cleaved HOPG surface were minutes with only a momentary rise of the base used in these studies. pressure. The sample temperature can be varied The images that are discussed were obtained from 77 K to 1500 K while being monitored with a at room temperature in air at varying tip scan chromeValumel thermocouple attached to the speeds, which are noted with each image. A tunnel­ sample. The instrumentation and data collection are ing current set point of less than 1 nA was used. A controlled using data acquisition and graphics differential input preamplifier, in which a "noise" programs written in this laboratory. The UHV input is electronically subtracted from a "signal plus chamber is shown schematically in Figure 2. noise" input, has recently been incorporated into the For the analyses presented in this paper, th experimental setup [J. Katz and the LBL STM group typical chamber pressure was less than 1 x 10-9 are credited for the design of this preamplifier and Torr, and the plane of the sample was analyzed its incorporation into the STM]. The "noise" input is normal to the entrance axis of the hemispherical ki­ connected to a wire which makes a path that is netic energy analyzer with the electron gun (AES) nearly identical to the path made by the "signal plus and the x-ray gun (ESCA) positioned at a 60" angle noise" wire leading to the tip. This "noise" wire ter­ with respect to the surface normal and working dis­ minates near the STM tip without making electrical tances of approximately 25 mm. The AES spectra contact. This preamplifier operates with a gain of were obtained in the derivative mode at a primary 0.10 nA/V on a ten volt full scale with a unity gain beam energy of 3000 eV and 5 V peak-to-peak mod­ bandwidth of approximately 2 kHz and a noise level ulation of the pass energy. The ESCA spectra were of approximately 2 pA peak-to-peak. The STM was obtained using both Mg(K ) and Al(K,.) sources at controlled using feedback, scanning, and offset elec­ anode powers ranging from ~40 W to 390'W (specifics tronics built at the University of Utah, and images are listed with data). were acquired using an 80386/387 based 20 MHz AT compatible computer system equipped with a 12-bit, 150 kHz analog-to-digital converter. Images consist Individual Sample Preparation of 256 x 256 arrays of 12-bit data obtained in the constant current imaging mode. Image figures were Biological Samples photographed from the computer screen. An 8-mer olW,onucleotide with a thiolated AES and ESCA analyses were performed in a guanine (5'-AAAC-S G-TTT-3') at a concentration of newly constructed ultrahigh vacuum (UHV) surface 100 µg/ml (in nanopure H20; residual counterions science chamber designed and built in this labora­ are present - see Verification Studies) was used in tory [manuscript in progress, Leavitt AJ, Han T, these experiments [12].
Details
-
File Typepdf
-
Upload Time-
-
Content LanguagesEnglish
-
Upload UserAnonymous/Not logged-in
-
File Pages16 Page
-
File Size-