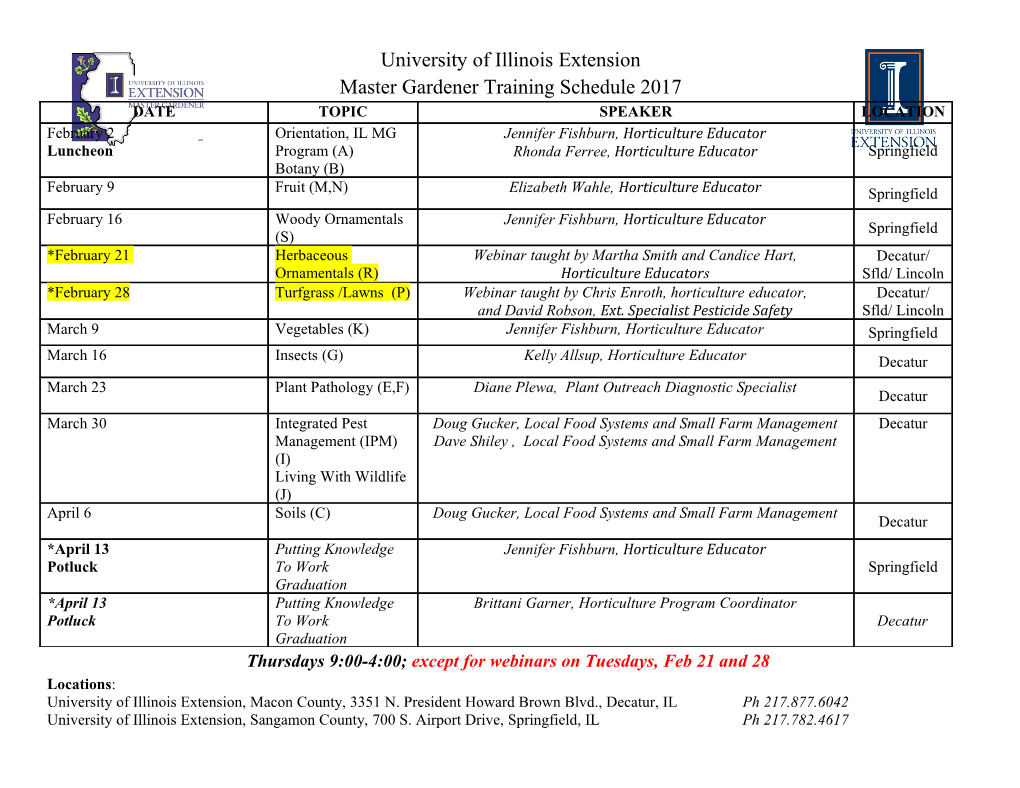
ENGINEERED TECHNOLOGIES TO STUDY THE ROLE OF MECHANICAL SIGNALS IN HUMAN LYMPHOMA GROWTH AND THERAPEUTIC RESPONSE A Dissertation Presented to the Faculty of the Graduate School of Cornell University In Partial Fulfillment of the Requirements for the Degree of Doctor of Philosophy by Apoorva May 2018 © 2018 Apoorva ACKNOWLEDGEMENT I am very thankful to my advisor Ankur for his mentorship, support and constant encouragement. His passion and enthusiasm for research are contagious and inspiring. I appreciate his engaging, hands-on and proactive approach to research. I also appreciate Ankur’s patience, and how he went out of his ways to help me on several occasions-- Anything good I do, will be because of him. All this would not have been possible without Marcia Sawyer. Thank you to Marcia for all her unconditional support, encouragement and help. I am glad that when PhD gets tough, Marcia is around to talk to. Last but not least, I appreciate how she kept all the grad students informed and organized. For the phosphorylation studies, Prof. Cosgrove and Alex made significant contribution to the experiments and analysis. I am grateful to Prof. Cosgrove for inspiring discussions, feedback and access to his lab facility. For the computational analysis and microfluidic experiments, I often visited Prof. Kirby. I thank him for his time and ideas. I am also grateful to my other committee members, Prof. Archer and Prof. Shepherd, for their feedback and guidance. When I joined Singh lab, I had absolutely zero knowledge of biomedical engineering. Field and Alberto were the only students in the lab. They were both colleagues as well as friends. Field and Alberto contributed immensely in getting me started from zero, and I am truly thankful to them. During next three years of joining the lab I witnessed Singh lab growing into the most diverse, energetic and fun lab. It was a pleasure to work with or alongside of Matt, Regan, Jessica and Shiv. I am especially grateful to Kristine for assisting me in countless number of assays and cultures. I also thank her for editing and feedback on first and fourth chapter of this dissertation. My interaction with the entrepreneurship community at Cornell has been a rewarding and enriching experience. I thoroughly enjoyed all the hackathons and summits organized by Cornell entrepreneurship community. Thank you to Ken Rother, Steven Gall, Brad Treat, Tom Schryver and Felix Litvinsky for teaching me on every step of this journey, and making this experience remarkable. Jason and Pankaj were my companions for everything. I cherish my true friendship with J&P, I appreciate them sticking with me through my thick and thin. This part of my Cornell experience would always hold a special place in my memory. This journey would also be memorable because of Abhishek, Rina, Igor, Ritesh and Pooja. The many interesting talks I had with Akanksha on random topics at random places in random hours were integral to this journey. Akanksha l, you were my companions through “ups and downs” and I cherish our little “roller coaster ride”. Ma, Papa, Mama and Tullu are always there for me with their unquestioned and unconditional support. As I always wonder- How could I thank them. I don’t know. Maybe I can’t. And so, I won’t. ENGINEERED TECHNOLOGIES TO STUDY THE ROLE OF MECHANICAL SIGNALS IN HUMAN LYMPHOMA GROWTH AND THERAPEUTIC RESPONSE Apoorva, Ph.D. Cornell University, 2018 Diffuse Large B cell lymphoma (DLBCL) is the most common lymphoma representing ~30% of all B cell Non-Hodgkin lymphomas. DLBCL is a heterogeneous disease associated with a variety of clinical presentations and genetic diversity (1). The standard combination chemotherapy, Rituximab (R)- CHOP (doxorubicin, vincristine, prednisone, mechlorethamine) has been the frontline therapy for years, and still a significant percentage of DLBCL patients are not cured (2). Activated B cell-like (ABC) DLBCL is the most chemo-resistant DLBCL subtype to R-CHOP with a 5-year overall survival as low as 40% vs 80% for germinal center B cell-like (GCB) DLBCL (1, 3). A myriad of independently predictive biomarkers for resistance have been identified, including gene expression signature, stromal signatures, epigenetic silencing of specific genes, and specific somatic mutations (3, 4). But none is sufficient to predict resistance in a given patient and few are helpful in guiding the selection of targeted therapies. Therefore, new treatments and treatment-specific biomarkers are needed to improve clinical outcome of ABC-DLBCL. It is becoming increasingly evident that tumor microenvironment is an active participant in the progression and pathogenesis of lymphoma (5, 6). DLBCLs originate and reside in lymphoid tissues subjected characteristic lymphatic and vascular fluid forces, extracellular matrices, and cell-cell interactions with a variety of stromal and immune cells. In the context of ABC-DLBCL, which are the most chemoresistant subtypes, the hallmark ABC-DLBCL mutations result in constitutive activation of B cell receptor (BCR) pathways. Hence these pathways are emerging as a source of therapeutic targets for the treatment of these tumors. However to date, existing BCR pathway inhibitors such as those targeting Bruton’s tyrosine kinase (BTK) are active in a limited subset of patients and only for a short duration (few months). Therefore, there is a need to understand factors that modulate BCR. My dissertation focuses on three components of lymphoma microenvironment, namely (a) fluid shear stress and mass transport, (b) tissue stiffness, and (c) vascularization. To study the role of lymphatics-mediatedshear stress in DLBCLs, we engineered an integrated cell culture micro-reactor platform with micron-scale high resistance channels that recapitulates fluid flow velocities, pressure, and shear stresses developed in subcapsular sinuses of lymph nodes. The findings suggest that lymphatic-grade shear stress increases DLBCL cell proliferation and reduces chemotherapeutic responsiveness across DLBCL subtypes. The interplay of α4β1-integrin, CD20, and B cell receptors guides DLBCLs to respond differentially to fluid shear stress and nutrient mass transport, by altering the phosphorylation of downstream signaling pathways. The dissertation next focuses on the role of mechanical stiffness of the malignant lymphoid tissues. The DLBCLs are commonly characterized by enlargement and palpable stiffness of lymph nodes. However, there are no studies on the quantification of tissue stiffness in lymphomas and on the role, it can play in tumor progression or therapeutic response. We first determined the strain energy density of freshly isolated healthy and tumorous mouse lymph nodes, as well as lymphoma tissue from a patient using a micropipette aspiration technique, previously applied to quantify the local stiffness measurement of soft tissues (7, 8). We next developed stiffness-matched hydrogels to elucidate the role of lymphoid tissue stiffness on proliferation, phenotype, and therapeutic response of DLBCLs. The final part of the dissertation focuses on a cellular component of DLBCL microenvironment – the endothelial cells, which play an important role in angiogenesis. It is now increasingly recognized that DLBCLs are promoted by two angiogenic modes, firstly by autocrine signals via self-expressed VEGFR and VEGF, and secondly by paracrine signals of endothelial progenitors of the microenvironment (9). In this dissertation we present an engineered bio-artificial organoid platform to study the interaction between DLBCL and endothelial cells, the angiogenic component of lymphoma microenvironment. We demonstrate that two integrins α4β1 and αvβ3, expressed by both DLBCLs and endothelial cells, modulate the VEGF, as well as CD20 and B cell receptor expressed by DLCBL cells. We anticipate that our engineered technology will be useful in study of lymphoma biology, and discovery and optimization of new class of therapeutics. Key word: Lymphoma, Organoids, Integrins, Micro-reactors, Angiogenesis REFERENCES: 1. Roschewski M, Staudt LM, Wilson WH. Diffuse large B-cell lymphoma- treatment approaches in the molecular era. Nat Rev Clin Oncol. 2014;11(1):12-23. Epub 2013/11/13. doi: 10.1038/nrclinonc.2013.197. PubMed PMID: 24217204. 2. Friedberg JW. Relapsed/refractory diffuse large B-cell lymphoma. Hematology Am Soc Hematol Educ Program. 2011;2011:498-505. Epub 2011/12/14. doi: 10.1182/asheducation-2011.1.498. PubMed PMID: 22160081. 3. Lenz G, Wright G, Dave SS, Xiao W, Powell J, Zhao H, Xu W, Tan B, Goldschmidt N, Iqbal J, Vose J, Bast M, Fu K, Weisenburger DD, Greiner TC, Armitage JO, Kyle A, May L, Gascoyne RD, Connors JM, Troen G, Holte H, Kvaloy S, Dierickx D, Verhoef G, Delabie J, Smeland EB, Jares P, Martinez A, Lopez-Guillermo A, Montserrat E, Campo E, Braziel RM, Miller TP, Rimsza LM, Cook JR, Pohlman B, Sweetenham J, Tubbs RR, Fisher RI, Hartmann E, Rosenwald A, Ott G, Muller- Hermelink HK, Wrench D, Lister TA, Jaffe ES, Wilson WH, Chan WC, Staudt LM, Lymphoma/Leukemia Molecular Profiling P. Stromal gene signatures in large-B-cell lymphomas. N Engl J Med. 2008;359(22):2313-23. doi: 10.1056/NEJMoa0802885. PubMed PMID: 19038878. 4. Sehn LH, Gascoyne RD. Diffuse large B-cell lymphoma: optimizing outcome in the context of clinical and biologic heterogeneity. Blood. 2015;125(1):22-32. doi: 10.1182/blood-2014-05-577189. PubMed PMID: WOS:000350809500009. 5. Scott DW, Gascoyne RD. The tumour microenvironment in B cell lymphomas. Nat Rev Cancer. 2014;14(8):517-34. Epub 2014/07/11. doi: 10.1038/nrc3774. PubMed PMID: 25008267. 6. Burger JA, Wiestner A. Targeting B cell receptor signalling in cancer: preclinical and clinical advances. Nat Rev Cancer. 2018;18(3):148-67. Epub 2018/01/20. doi: 10.1038/nrc.2017.121 nrc.2017.121 [pii]. PubMed PMID: 29348577. 7. Aoki T, Ohashi T, Matsumoto T, Sato M. The pipette aspiration applied to the local stiffness measurement of soft tissues. Ann Biomed Eng. 1997;25(3):581-7. Epub 1997/05/01. PubMed PMID: 9146811.
Details
-
File Typepdf
-
Upload Time-
-
Content LanguagesEnglish
-
Upload UserAnonymous/Not logged-in
-
File Pages168 Page
-
File Size-