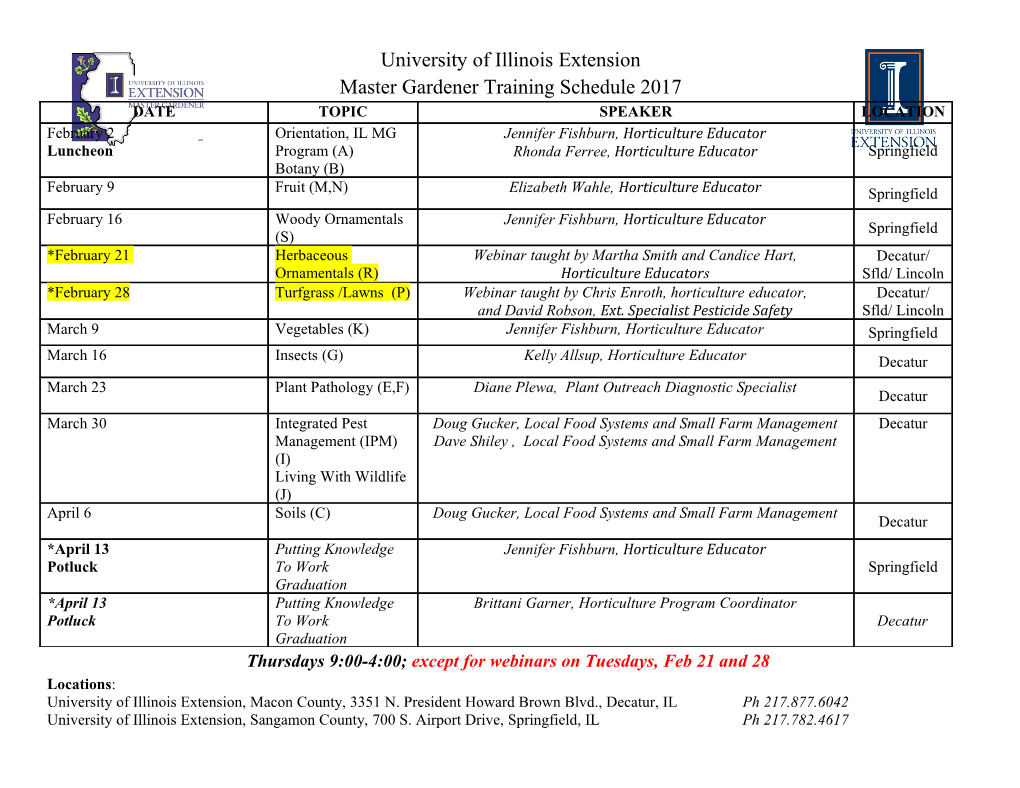
Adam Johnson Department of Molecular and Cellular Biochemistry Lisa M. Pratt Department of Geological Sciences Indiana University Bloomington, Indiana Introduction Recent activity on Mars has rekindled thoughts of life on the surface and subsurface Thus far, the search for organic carbon and biomarkers on Mars yielded negative results y Active oxidation process y Hypothesized oxidants include: ○ Photochemical ○ Ionizing radiation ○ Peroxides and oxides/hydroxides ○ Chemical reactivity of the regolith (Fenton) Work must be done to determine cumulative effects, primary mechanisms and kinetics of organic matter oxidation Experimental Goals Perform long term simulation study with accurate mimicking of Mars surface conditions and regolith composition Investigate the cumulative effects of photochemical and regolith reactivity on biomarker oxidation y Investigate the effects of depth below the profile surface y Correlate oxidation kinetics with various reaction pathways (UV, peroxide, Fenton oxidation) What Biomarker? Dextrorotary Alanine Levorotary Alanine 50% dry cell weight Moderately good preservation potential Optical activity differentiates from abiotic forms Indiana Mars Analog Regolith Simulant-2 (I-MARS-2) Holocene andesitic basalt w/ olivine Various iron oxides, phyllosilicates, iron sulfates and sulfate salts Mars Simulated Atmospheric Composition Mars Simulated Solar Spectrum Mars Diurnal Cycle Experimental Layout Quartz tubes ~1.0 gram regolith with ~3.0 cm depth profile y Subsampled at 1.0 cm depth profiles 100 ppm of L-Asp, L-Glu, L- Ala, L-Val and Gly Three sample categories y Mars conditions with UV y Mars conditions w/o UV y Controls at room temp and RH Triplicate samples removed at 0, 10, 20, 30 and 40 days. Results Acid hydrolysis, desalting, separation via HPLC as their OPA/NAC derivatives with UV detection Retention Time Rapid drop in the top of UV irradiated samples (red) y Small change in middle and bottom profiles until T=3 Dark samples (blue) show minimal/no change at T=1 and increase with depth to plateau near -100 ppm; largest values at bottom. Control samples (green) show minimal loss in the bottom layer until after T=2; maximum loss occurring in the top and middle layers. -kt Nt=N0 e First order kinetics Obvious trends in oxidation rate data; y UV samples show top down decrease y Dark samples show bottom up decrease y Controls show rate constant fairly independent; maybe increase in middle Oxidation Rates Converge as they Approach Bottom; Similar Oxidation Mechanism Below the Top Centimeter? Conclusions Data indicates multiple oxidation mechanisms y Photochemical oxidation in upper cm of UV sample ○ Only accounts for irradiation of about 0.1% of surface area; the remaining amount must be something else y Water increases rates of oxidation several orders of magnitude ○ Oxide radicals (Yen, 2000); peroxides (Bullock, 1994); hydroxide radicals (Benner, 2000) through metal leaching So increased water increased oxidation! Oxidation mechanisms that are controlled at depth by the rate of diffusion of water through the sample Dark Samples •Low temp; water in regolith freezes UV •Upper and middle Samples layers rapidly •Higher Temp sublimate; re-saturate (radiative heat) with time with •Water in regolith downward diffusion rapidly vaporizes away Control •UV in the upper layer Samples rapidly oxidizes organics •MUCH higher temp; •Diffusion of rapid diffusion and water/peroxides exchange of water through regolith with between regolith and time atmosphere from all depths Conclusions A two part oxidation mechanism y A photolytic component in UV irradiated samples gives rise to rapid oxidation y With time and in a depth dependent manner, the diffusion of water and/or products from water photolysis diffusion through the regolith y Increased oxidation of the Martian regolith is due to the interaction of even minor amounts of water with minerals on the Martian surface Missions designed to search for organic carbon/signs of life on Mars must sample from below this diffusion boundary! Acknowledgements Indiana Princeton Tennessee Astrobiology Institute y Lisa Pratt TechShot Laboratories of Greenville, IN Collaborators from several universities y Tullis Onstott, Princeton y Rocco Mancinelli, SETI y Lynn Rothschild, NASA Ames y Susan Pfiffner, Tennessee y Lyle White, McGill University y Gaetan Borgonie, University of Ghent y Ruth Bryant, Albert Einstein School of Medicine Indiana Space Grant References Benner, S. A, et al. 2000. PNAS. 97, 2425-2430. Biemann, K., et al. 1977. J. Geophys. Res. 82, 4641- 4658. Bullock, M. A.,et al. 1994. Icarus. 107, 142-154. Chun, S. F. S., et al. 1978. Nature. 274, 875-876. Garry, J. R. C., et al. 2006. MAPS. 41, 391-405. Kminek, G., Bada, J. L., 2006. EPSL. 245, 1-5. McDonald, G. D., et al. 1998. Icarus. 132, 170-175. Mumma, M. J., et al. 2009. Science. 1041-1045. Yen, A. S., et al. 2000. Science. 289, 1909-1912. Zent, A. P. McKay, C. P., 1994. Icarus. 108, 146- 157..
Details
-
File Typepdf
-
Upload Time-
-
Content LanguagesEnglish
-
Upload UserAnonymous/Not logged-in
-
File Pages17 Page
-
File Size-