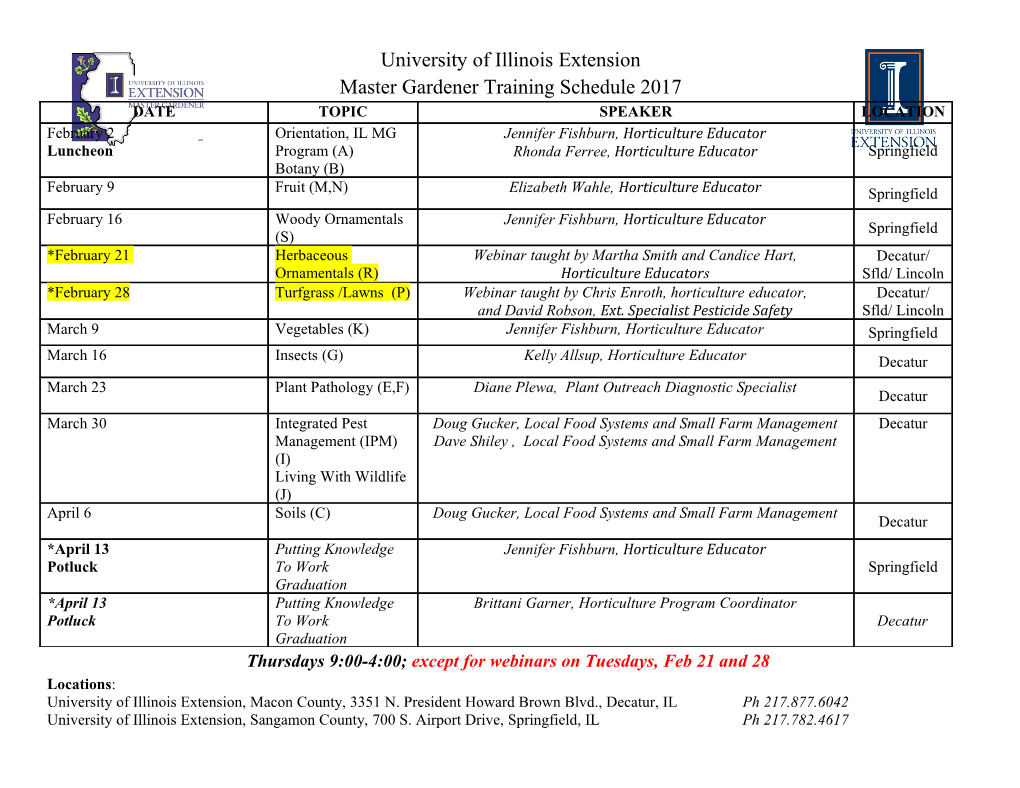
catalysts Article Synthesis of Tetrahydropyran from Tetrahydrofurfuryl Alcohol over Cu–Zno/Al2O3 under a Gaseous-Phase Condition Fengyuan Zhang 1,2,3, Bin Zhang 4 ID , Xincheng Wang 1,2, Long Huang 1,2,*, Dekun Ji 1,2, Songsong Du 1,3, Lei Ma 1,2 and Shijing Lin 1,2 1 College of Chemical Engineering, Beijing Institute of Petrochemical Technology, Beijing 102617, China; [email protected] (F.Z.); [email protected] (X.W.); [email protected] (D.J.); [email protected] (S.D.); [email protected] (L.M.); [email protected] (S.L.) 2 Beijing Key Laboratory of Fuels Cleaning and Advanced Catalytic Emission Reduction Technology, Beijing 102617, China 3 Graduate School of Beijing University of Chemical Technology, Beijing 100029, China 4 Institute of Coal Chemistry, Chinese Academy of Sciences, P.O. Box 165, Taiyuan 030001, China; [email protected] * Correspondence: [email protected]; Tel.: +86-010-8129-2131 Received: 24 January 2018; Accepted: 7 February 2018; Published: 6 March 2018 Abstract: Tetrahydropyran (THP) represents an O-containing hetero-cyclic compound that can be used as a promising solvent or monomer for polymer synthesis. In this work, Cu–ZnO/Al2O3 catalysts have been prepared by a facile precipitation–extrusion method and used for the synthesis of THP through gaseous-phase hydrogenolysis of tetrahydrofurfuryl alcohol (THFA). The effect of the molar ratio of Cu/Zn/Al, reaction temperature, and hydrogen pressure was investigated. An 89.4% ◦ selectivity of THP was achieved at 270 C and 1.0 MPa H2. Meanwhile, the optimum molar ratio of Cu/Zn/Al was determined to be 4:1:10. The Cu–ZnO/Al2O3 catalyst exhibited high catalytic activity and stability for 205 h on-stream. A possible reaction mechanism involving several consecutive reactions was proposed: THFA was firstly rearranged to 2-hydroxytetrahydropyran (2-HTHP), followed by the dehydration of 2-HTHP to 3,4-2H-dihydropyran (DHP) over acid sites; finally, the DHP was hydrogenated to THP. The synergy of acid sites and metal sites of Cu–ZnO/Al2O3 played an important role during the production of THP. Keywords: tetrahydropyran; tetrahydrofurfuryl alcohol; Cu–ZnO/Al2O3 1. Introduction Catalytic upgrading of biomass-derived furfural or its derivatives to value-added chemicals has received great attention in recent years [1–3]. Furfural and 5-hydroxymethylfurfural (HMF), which are considered to be promising biorenewable platform chemicals, can be derived through acid-catalyzed dehydration of hemicellulose from various abundant agricultural raw materials, such as hemicellulose grain shell, hardwood lumber, corncobs, wheatbran, and other renewable biomass materials [4–7]. There were intense studies carried out on the production of high value-added chemical intermediates and end products from HMF using heterogeneous catalysts [8–11]. Tetrahyrofurfuryl alcohol (THFA) can be obtained through hydrogenation of furfural at a very high yield [12], and from which various useful chemicals, such as 1,5-pentadiol (15PDO) [13–25], 3,4-2H-dihydropyran (DHP) [26–28], and 4-penten-1-ol can be produced [29,30]. 15PDO, via a ring closure pathway, can form tetrahydropyran (THP) through the participation of strong solid acid catalysts or high-temperature water [31]. Moreover, THP can be obtained by the hydrogenation of DHP [32]. THP can be used as both a solvent and an intermediate in the synthesis of organic compounds such as glutaric acid, Catalysts 2018, 8, 105; doi:10.3390/catal8030105 www.mdpi.com/journal/catalysts Catalysts 2018, 8, 105 2 of 11 heptanediamine, 1,5-dichloropentane, and pimelic acid [32,33]. However, the synthesis of THP directly from THFA using heterogeneous catalysts is rarely reported. Recently, the catalytic conversion of furanics has been extensively studied over noble metal catalysts. However, due to the high cost and depleting resources of noble metals, it is of great importance to explore catalytic systems over non-noble metals. Among non-noble metals, copper-based catalysts were widely used in hydrogenation and hydrogenolysis reactions due to their good catalytic performance and low cost. Müller et al. [34] found that dimethyl succinate could be completely converted to THF over CuO–ZnO/Al2O3 at 220/Allely MPa. Guo et al. [35] also reported that a selectivity of THF as high as 94% could be obtained over Cu-B/Al2O3 during the hydrogenolysis of dimethylsuccinate. The selective conversion of furanic-derived compounds over Cu catalysts is particularly encouraging and needs further investigation. Soghrati et al. [36] reported that a 70% yield of THP was achieved via hydrogenolysis of THFA over Ni/HZSM-5 catalyst, encouraging us to investigate the probability of producing THP from THFA over Cu catalysts. In this paper, we have prepared a bi-functional Cu–ZnO/Al2O3 catalyst by a facile precipitation extrusion method. The physic-chemical properties were characterized by XRD, BET, TEM, NH3-TPD, and H2-TPR analysis. The role of Cu metal sites during the direct conversion of THFA to THP over Cu–ZnO/Al2O3 catalysts was studied. The effect of temperature, pressure, and catalysts on THFA to THP along with side reactions was investigated. The current work was beneficial to develop the downstreams of TFHA over non-noble catalysts. 2. Results and Discussion 2.1. Catalyst Characterization 2.1.1. BET Characterization The porosity and pore-size distribution of the calcined Cu–ZnO/Al2O3 extrudate catalysts were determined by N2 adsorption–desorption. The γ-Al2O3 (boehmite particles behaving as a binder and supplying acid sites) exhibited a 12 nm mean diameter (Figure S1). Accordingly, the volume-size distribution of Cu–Zn particles was characterized as a bimodal distribution with a mean diameter of 2.7 nm. The other peak resulting from 8.2 nm particles was possibly derived from symbiotic fragments. Therefore, the extrudate’s pore size distribution might be related with the solid composition, because the respective catalysts particle sizes differed significantly; therefore, the effect of the amount of γ-Al2O3 on the main peak of the size distribution was not negligible. Lamelleted mesoporous materials exhibited a typical type-III shaped isotherm, obtained by nitrogen adsorption–desorption measurements (Figure S2). The surface areas (SBET) of the calcined catalysts 2 −1 increased monotonically from 196.4 to 261.7 m ·g with the increase in the content of γ-Al2O3, though they were generally lower than that of γ-Al2O3 (A0-0-01) (Table1). This is consistent with the study by Kraushaar-Czarnetzki et al. [34]. Table 1. The physicochemical properties of the catalysts. Cu/Zn/Al Pore Volume Copper Crystalline Average Pore Catalysts SBET (m2·g−1) Molar Ratio (cc·g−1) Size (nm) Size (nm) A4-1-00 4:1:00 41.3 0.18 34.4 8.8 A0-0-01 0:0:10 310.3 0.94 - 6.1 A4-1-03 4:1:03 196.4 0.54 26.6 4.7 A4-1-05 4:1:05 199.3 0.61 25.8 4.5 A4-1-07 4:1:07 203.5 0.63 25.0 3.9 A4-1-10 4:1:10 223.8 0.68 24.3 3.8 A4-1-15 4:1:15 261.7 0.70 22.3 3.6 Catalysts 2018, 8, 105 3 of 11 Catalysts 2018, 8, x FOR PEER REVIEW 3 of 11 2.1.2. XRD Characterization The XRD patterns of calcined catalysts are shownshown inin FigureFigure1 .1. DiffuseDiffuse diffractiondiffraction peakspeaks atat 2θ θ = 35.5°, 35.5◦, 38.9°, 38.9◦, and and 64.2° 64.2◦ (JCPDS(JCPDS 05 05-0661)‐0661) were were attributed attributed to to crystalline crystalline CuO, and the XRD patterns were associatedassociated with with the the copper copper crystalline crystalline size size and and the the copper copper species species [37]. [37]. The The peak peak intensities intensities of both of bothCuO andCuO ZnO and wereZnO weakened were weakened with the with increase the increase in aluminum in aluminum content, possiblycontent, duepossibly to the due increased to the increaseddispersion dispersion of Cu and Znof speciesCu and after Zn thespecies addition after of theγ-Al addition2O3 (Figure of γ‐2).Al The2O3 copper(Figure crystallite 2). The copper size of crystalliteall catalysts size were of determinedall catalysts towere be 34.4determined nm, 26.6 to nm, be 25.834.4 nm,nm, 25.026.6 nm, nm, 24.3 25.8 nm, nm, and 25.0 22.3 nm, nm 24.3 by nm, the ◦ andScherrer 22.3 equation,nm by the based Scherrer on the equation, full width based at maximumon the full Cu width diffraction at maximum of 35.5 Cu. The diffraction peak of γof-Al 35.5°.2O3 wasThe peak not observed of γ‐Al2O due3 was to thenot highobserved dispersion due to of theγ-Al high2O dispersion3 species. of γ‐Al2O3 species. A0-0-10 A4-1-15 A4-1-10 A4-1-07 A4-1-05 A 4-1-03 Intensity (a.u) CuO :ZnO A4-1-00 20 30 40 50 60 70 80 2Theta Figure 1. XRD patterns of calcined catalysts samples with different amount of γ‐Al2O3. Figure 1. XRD patterns of calcined catalysts samples with different amount of γ-Al2O3. 2.1.3. NH3‐TPD Characterization 2.1.3. NH3-TPD Characterization The acidity of samples was determined by NH3‐TPD. The acid distribution was obtained by The acidity of samples was determined by NH3-TPD. The acid distribution was obtained by Gaussian fitting of NH3‐TPD curves (Table 2 and Figure S3). Three peaks centered at 104 °C,◦ 225 °C,◦ Gaussian fitting of NH3-TPD curves (Table2 and Figure S3). Three peaks centered at 104 C, 225 C, and 335 ◦°C were fitted for the NH3‐TPD curve, attributing to the NH3 desorption from the weak, and 335 C were fitted for the NH3-TPD curve, attributing to the NH3 desorption from the weak, medium, and strong acid sites, respectively [38].
Details
-
File Typepdf
-
Upload Time-
-
Content LanguagesEnglish
-
Upload UserAnonymous/Not logged-in
-
File Pages11 Page
-
File Size-