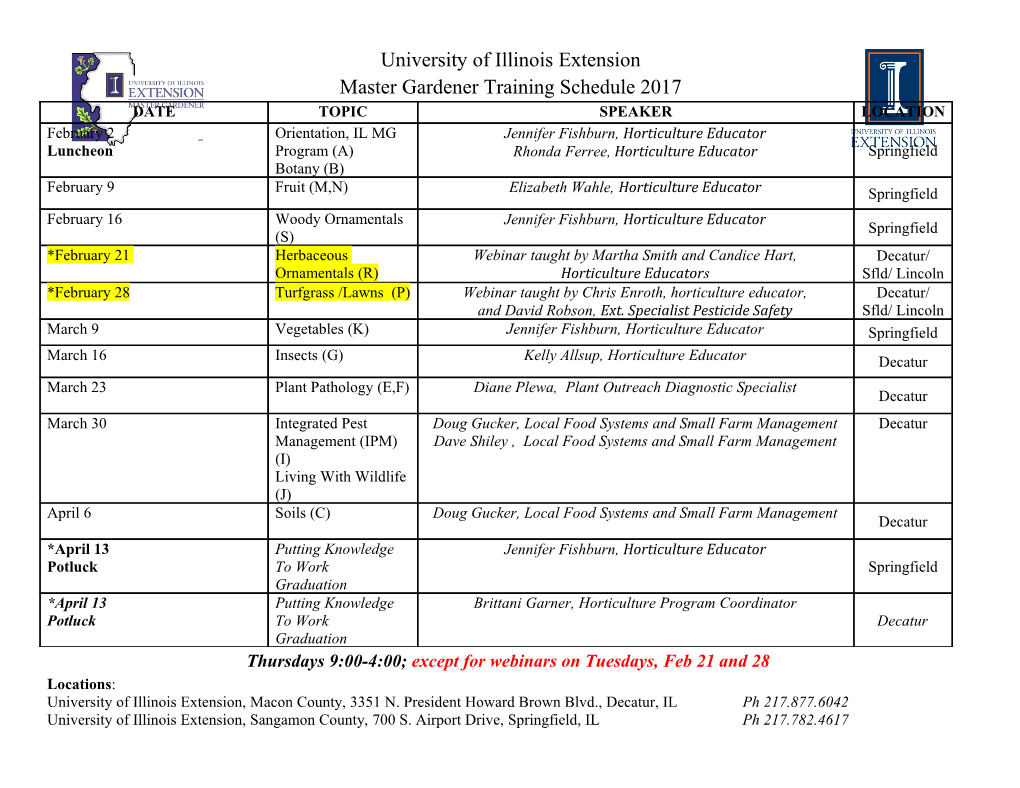
Journal of Structural Geology 32 (2010) 855–875 Contents lists available at ScienceDirect Journal of Structural Geology journal homepage: www.elsevier.com/locate/jsg Review Article Interpretation and analysis of planetary structures Richard A. Schultz a,*, Ernst Hauber b, Simon A. Kattenhorn c, Chris H. Okubo d, Thomas R. Watters e a Geomechanics-Rock Fracture Group, Department of Geological Sciences and Engineering/172, University of Nevada, Reno, NV 89557-0138, United States b DLR-Institut fu¨r Planetenforschung, Rutherfordstrasse 2, D-12489 Berlin-Adlershof, Germany c Department of Geological Sciences, University of Idaho, P.O. Box 443022, Moscow, ID 83844-3022, United States d U.S. Geological Survey, 2255 North Gemini Drive, Flagstaff, AZ 86001, United States e Center for Earth and Planetary Studies, National Air and Space Museum, Smithsonian Institution, Washington, DC 20560, United States article info abstract Article history: Structural geology is an integral part of planetary science. Planetary structures provide the framework for Received 24 April 2009 determining the character and sequence of crustal deformation while simultaneously establishing the Received in revised form observational basis required to test geodynamic hypotheses for the deformation of planetary and satellite 31 August 2009 lithospheres. The availability of datasets that record spatial and topographic information with a resolution Accepted 13 September 2009 that matches or, in many cases, exceeds, what is available for Earth-based studies permits the deformation Available online 11 December 2009 of several planets and satellites to be investigated down to the local or outcrop scales. The geometry and kinematics of common planetary structures such as joints, igneous dikes, deformation bands, faults, and Keywords: Planetary structural geology folds can be determined with confidence from their distinctive morphologic and topographic signatures, Faulting enabling the structural histories and deformation magnitudes to be determined. Segmentation, displace- Folding ment profiles, relay ramps, footwall anticlines, displacement-controlled depocenters, and other well- Deformation bands known characteristics of terrestrial normal fault and graben systems reveal the sequence and processes of Joints fault growth in numerous planetary examples. Systems of thrust faults having both blind and surface- Fracture mechanics breaking components are important elements on several bodies including Mercury, the Moon, and Mars. Strike-slip faults have been identified on bodies including Mars and Europa with oblique extension found on Ganymede. Using field-based studies of Earth-based structures as a guide, planetary structures provide a means to explore and evaluate the causative stresses. Despite the wide range in structural styles across the solar system, plate tectonics is recognized only on the Earth, with the other planets and satellites deforming in the absence of large-scale horizontal motions and attendant plate recycling. Ó 2010 Elsevier Ltd. All rights reserved. 1. Introduction and Bulmer, 1998; Schultz, 2000a; Okubo and Schultz, 2004). Arcuate fold belts related to contractional strain have been identi- Deformation of the lithospheres of planets and satellites has fied in the icy lithospheric shell of Enceladus (Porco et al., 2006), produced populations of structures that appear to be strikingly and rare folding has also been inferred on Europa (Prockter and similar to those found on Earth (see Watters and Schultz, 2010, for Pappalardo, 2000). Strike-slip faults have been identified on Mars a comprehensive overview), both morphologically and mechan- (e.g., Schultz, 1989; Okubo and Schultz, 2006a; Andrews-Hanna ically. Faults in particular have been documented on nearly every et al., 2008) and the icy satellites Europa (Schenk and McKinnon, geologic surface in the solar system, occurring in both lower-strain 1989; Hoppa et al., 1999a; Kattenhorn, 2004; Kattenhorn and distributed and higher-strain localized regimes. Normal faults and Marshall, 2006) and Ganymede (in association with normal fault- grabens are found on Mercury, Venus, the Moon, Mars, and icy ing; DeRemer and Pappalardo, 2003; Pappalardo and Collins, 2005). satellites of the outer planets such as Europa, Ganymede, Miranda, Individual dilatant cracks (joints; Schultz and Fossen, 2008)have Ariel, Dione, Tethys, Rhea, and Titania (e.g., Watters and Schultz, been identified on Mars (Okubo and McEwen, 2007; Okubo et al., 2010). Thrust faults have been recognized on Mercury, Venus, the 2009) and are pervasive on icy moons of the outer solar system Moon, Mars, and Io along with their surficial anticlines, called such as Europa (Figueredo and Greeley, 2000, 2004; Kattenhorn, wrinkle ridges (Plescia and Golombek, 1986; Watters, 1988; Schenk 2002; Marshall and Kattenhorn, 2005) and Enceladus (Kargel and Pozio, 1996; Porco et al., 2006). Deformation bands (Aydin et al., 2006; Fossen et al., 2007) have been identified on Mars (Okubo and * Corresponding author. McEwen, 2007; Okubo et al., 2009) and have been suggested to E-mail address: [email protected] (R.A. Schultz). occur on Europa (Aydin, 2006). The presence of subsurface igneous 0191-8141/$ – see front matter Ó 2010 Elsevier Ltd. All rights reserved. doi:10.1016/j.jsg.2009.09.005 856 R.A. Schultz et al. / Journal of Structural Geology 32 (2010) 855–875 dikes has been inferred on Mars from surface topographic data (MDIS) will provide a 250-m per pixel or better global mosaic, and (Schultz et al., 2004) and, in this paper, identified there in high- the planet’s topography will be measured by the Mercury Laser resolution imaging data. Altimeter (MLA) instrument and using digital elevation models In this paper we gather and present some of the findings from derived from stereo images (Solomon et al., 2007, 2008). recent spacecraft exploration of the solid-surface planets and satellites in our solar system (see Beatty et al. (1999) for general 2.2. Moon information on the planets and satellites in our solar system). Following current usage, terrestrial planets are bodies having solid Several current and forthcoming datasets will complement the silicate crusts and include Mercury, Venus, Earth, Earth’s Moon, and high-quality imaging datasets acquired during the Apollo era of the Mars. Icy satellites are those whose crusts are primarily composed 1960s to early 1970s (e.g., Schultz, 1976; Masursky et al., 1978) of ices of water, methane, and ammonia and include most of the including Lunar Orbiter and Apollo metric and panoramic camera satellites of Jupiter, Saturn, Uranus and Neptune. For brevity we images (Tanaka et al., 2010) and images and data returned more refer the reader to McGill et al. (2010) for the structural geology of recently by the Clementine and Lunar Prospector missions. The Venus and to Collins et al. (2010) for studies of faulting and Lunar Reconnaissance Orbiter (LRO; Chin et al., 2007) has a suite of deformation of Ganymede, Callisto, and Io. We also do not discuss science instruments, two of which will be especially useful for the rather extensive literature on the structural geology of terres- investigating geologic structures. The Lunar Reconnaissance trial impact craters (see Earth Impact Database, 2007) despite its Orbiter Camera (LROC) is acquiring images with resolutions as high importance to the understanding of deformation processes on the as 0.5 m/pixel, with image footprints of typically 5 Â 25 km at an Earth and other planets (e.g., Laney and Van Schmus, 1978; Price altitude of 50 km. The Lunar Orbiter Laser Altimeter (LOLA) is and Cosgrove, 1990, pp. 112–118; Kriens et al., 1999; Huntoon, acquiring five simultaneous 5-m-diameter range measurements 2000; Kenkmann, 2002; Kenkmann et al., 2005; Pati and Reimold, per shot, with an along-track shot-to-shot spacing of 25 m. Data 2007). from LROC and LOLA are expected to be useful for investigating First we describe the principal types of data, such as imaging or geologic structures over a broad range of length scales. topography, that are being used to identify and interpret planetary structures (i.e., on planets and satellites other than the Earth). Next, 2.3. Mars we present a suite of results from the geologic mapping and anal- ysis of structures such as faults, folds, joints, and igneous dikes on The surface of Mars has been imaged since the flybys of Mariner bodies as diverse and complex as Mercury, the Moon, Mars, and icy 4 in 1965 and Mariners 6 and 7 in 1969, with global imaging satellites of Jupiter. Last, we explore two avenues that these acquired by the Mariner 9 orbiter in 1971 (see Carr,1980, for review). structures can provide into the mechanics of deformation on these The Viking 1 and 2 orbiters and landers further refined under- bodies. The overall theme of this paper is to demonstrate that standing of the nature of the Martian surface as well as providing an structural geology should not be considered to be restricted to the additional global dataset for the study of Martian structures (e.g., Earth, and that by studying other bodies with different geodynamic Arvidson et al., 1980). Since then many other US spacecraft, espe- styles we can learn about the response of lithospheres to a variety cially Mars Global Surveyor in 1999 (e.g., Smith et al., 2001), have of stress states having different origins. visited the planet and acquired high-resolution imaging, spectral, and topographic data of interest to structural and tectonic studies. 2. Data sets The Mars Orbiter Camera
Details
-
File Typepdf
-
Upload Time-
-
Content LanguagesEnglish
-
Upload UserAnonymous/Not logged-in
-
File Pages21 Page
-
File Size-