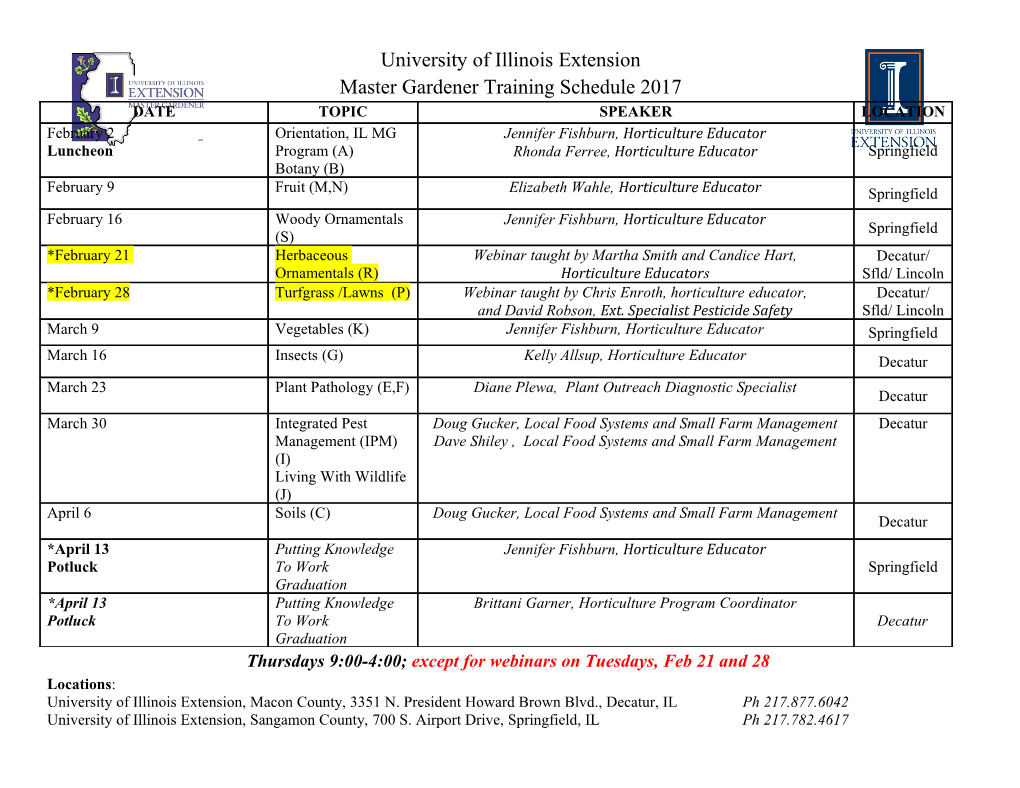
Crystal structure of elongation factor P from Thermus thermophilus HB8 Kyoko Hanawa-Suetsugu*, Shun-ichi Sekine†, Hiroaki Sakai*, Chie Hori-Takemoto*, Takaho Terada*†, Satoru Unzai*‡, Jeremy R. H. Tame*‡, Seiki Kuramitsu†§, Mikako Shirouzu*†, and Shigeyuki Yokoyama*†¶ʈ *RIKEN Genomic Sciences Center, 1-7-22 Suehiro-cho, Tsurumi, Yokohama 230-0045, Japan; †RIKEN Harima Institute at SPring-8, 1-1-1 Kouto, Mikazuki-cho, Sayo, Hyogo 679-5148, Japan; ‡Protein Design Laboratory, Yokohama City University, 1-7-29, Suehiro-cho, Tsurumi, Yokohama 230-0045, Japan; §Department of Biology, Graduate School of Science, Osaka University, Toyonaka, Osaka 560-0043, Japan; and ¶Department of Biophysics and Biochemistry, Graduate School of Science, University of Tokyo, 7-3-1 Hongo, Bunkyo-ku, Tokyo 113-0033, Japan Edited by Paul R. Schimmel, The Scripps Research Institute, La Jolla, CA, and approved May 13, 2004 (received for review December 26, 2003) Translation elongation factor P (EF-P) stimulates ribosomal pepti- other hand, hypusine is not found in bacteria (13). The structures dyltransferase activity. EF-P is conserved in bacteria and is essential of eIF-5A from three Archaea, Methanococcus jannaschii, Py- for cell viability. Eukarya and Archaea have an EF-P homologue, robaculum aerophilum, and Pyrococcus horikoshii, have two eukaryotic initiation factor 5A (eIF-5A). In the present study, we domains, which are composed of several -strands (14–16). determined the crystal structure of EF-P from Thermus thermophi- Crystallizations of EF-P from E. coli and Aquifex aeolicus have lus HB8 at a 1.65-Å resolution. EF-P consists of three -barrel been reported (7, 17). In this article, we report the crystal domains (I, II, and III), whereas eIF-5A has only two domains (N and structure of EF-P from Thermus thermophilus HB8 at a 1.65-Å C domains). Domain I of EF-P is topologically the same as the N resolution. The EF-P structures are -rich and is divided into domain of eIF-5A. On the other hand, EF-P domains II and III share three -barrel domains (domains I, II, and III). Domains II and the same topology as that of the eIF-5A C domain, indicating that III of EF-P share a very similar topology. The structures of domains II and III arose by duplication. Intriguingly, the N-terminal domains I and II of EF-P are superposable on the structures of half of domain II and the C-terminal half of domain III of EF-P have the M. jannaschii, P. aerophilum, and P. horikoshii eIF-5A sequence homologies to the N- and C-terminal halves, respectively, proteins. The overall structure of EF-P is strikingly similar to the of the eIF-5A C domain. The three domains of EF-P are arranged in L-shaped structure of tRNA. BIOCHEMISTRY an ‘‘L’’ shape, with 65- and 53-Å-long arms at an angle of 95°, which is reminiscent of tRNA. Furthermore, most of the EF-P protein Materials and Methods surface is negatively charged. Therefore, EF-P mimics the tRNA Protein Preparation and Crystallization. The DNA fragment encod- shape but uses domain topologies different from those of the ing EF-P, the protein TT0860 (DNA Data Base in Japan, known tRNA-mimicry translation factors. Domain I of EF-P has a accession no. AB103477), was isolated from the T. thermophilus conserved positive charge at its tip, like the eIF-5A N domain. HB8 genome and was cloned into the expression plasmid, pET11a (Novagen). E. coli BL21(DE3) was transformed with the ranslation elongation factor P (EF-P) was found as a protein vector, and T. thermophilus EF-P was overexpressed. The protein Tthat stimulates the peptidyltransferase activity of the 70S was purified by successive chromatography steps on Q Sepharose ribosome in Escherichia coli (1). EF-P enhances dipeptide and HiLoad Superdex 75 columns (Amersham Biosciences). synthesis with N-formylmethionyl-tRNA and puromycin in vitro, Hampton Research Crystal Screen (18) was used to determine suggesting its involvement in the formation of the first peptide the initial crystallization conditions for EF-P. The final crystal- bond of a protein (2). E. coli EF-P is encoded by the efp gene and lization conditions, 100 mM Hepes-Na buffer (pH 7.6) and 1.35 consists of 188 amino acid residues (3). The efp genes are M lithium sulfate at 16°C yielded high-quality crystals suitable universally conserved in Bacteria (4). Gene interruption exper- for x-ray diffraction data collection. They belong to the space ϭ ϭ iments in E. coli revealed that the efp gene is essential for cell group P212121, with unit cell dimensions a 55.8, b 78.4, and viability and is required for protein synthesis (3). The amount of c ϭ 138.9 Å. The crystallographic asymmetric unit contains two Ϸ ͞ EF-P in E. coli cells is 1 10th of that of EF-G; 800–900 nearly identical EF-P monomers. molecules of EF-P exist in a cell, an amount consistent with 1 EF-P per 10 ribosomes (5). Data Collection and Structure Determination. The crystal structure EF-P reportedly binds to both the 30S and 50S ribosomal of EF-P was solved by the multiple isomorphous replacement subunits (6). Antibiotic sensitivity and footprinting studies have method. Three heavy-atom derivatives were prepared by soak- indicated that EF-P binds near the streptomycin-binding site of ing the EF-P crystals for 12 h in reservoir solutions containing the 16S rRNA in the 30S subunit (6). EF-P also interacts with 2 mM potassium tetrachloroaurate (III), 2 mM sodium ethyl- domains II and V of the 23S rRNA, i.e., near the peptidyltrans- mercurithiosalicylate, and 2 mM mersalyl acid, respectively ferase center (PTC) (6, 7). Ribosome reconstitution experiments (Table 1). All the native and heavy-atom derivative data sets have shown that the L16 ribosomal protein or its N-terminal were collected from frozen crystals at 90 K by using synchro- 47-residue fragment was required for EF-P-mediated peptide tron radiation at the SPring-8 beam lines (Hyogo, Japan). The bond synthesis, whereas L11, L15, or L7͞L12 were not (8–10). data were processed with the program HKL2000 (19). Deter- Eukarya and Archaea seem to lack EF-P, although a similar mination of the heavy atom positions and calculation of the function may be mediated by eukaryotic initiation factor 5A (eIF-5A) (4, 6). The eIF-5A protein is composed of Ϸ140 amino Ϸ acid residues and is shorter than EF-P by 40 residues. Com- This paper was submitted directly (Track II) to the PNAS office. plete intracellular depletion of eIF-5A results in cell growth Abbreviations: EF, elongation factor; eIF-5A, eukaryotic initiation factor 5A; rmsd, rms inhibition; however, protein synthesis seems to be only slightly deviation. reduced (11). eIF-5A is a unique cellular protein that contains Data deposition: The atomic coordinates and structure factors have been deposited in the the unusual amino acid hypusine [N -(4-aminobutyl-2-hydroxy)- Protein Data Bank, www.pdb.org (PDB ID code 1UEB). L-lysine], which is formed by posttranslational modification of a ʈTo whom correspondence should be addressed. E-mail: [email protected]. specific lysine residue. The enzyme that modifies lysine to ac.jp. hypusine in eIF-5A is essential for yeast viability (12). On the © 2004 by The National Academy of Sciences of the USA www.pnas.org͞cgi͞doi͞10.1073͞pnas.0308667101 PNAS ͉ June 29, 2004 ͉ vol. 101 ͉ no. 26 ͉ 9595–9600 Downloaded by guest on October 1, 2021 Table 1. Crystallographic data Native K2AuCl4 EMTS Mersaryl acid Data collection Resolution,* Å 50–1.65 (1.71–4.65) 50–2.37 (2.45–2.37) 50–2.11 (2.19–2.11) 50–2.11 (2.19–2.11) Observed reflections, n 451,416 103,399 133,338 156,921 Unique reflections, n 62,691 21,379 30,131 30,123 Completeness,† % 99.9 (100.0) 98.8 (92.6) 99.9 (99.8) 99.4 (98.8) ‡ Rsym, % 7.7 (49.6) 3.4 (9.1) 5.5 (14.6) 8.1 (26.9) I͞(I)§ 26.4 (3.0) 44.9 (22.1) 26.4 (7.9) 18.9 (5.9) Heavy atom refinement and phasing Heavy atom sites, n 111 ¶ Riso, % 6.5 15.0 24.9 Phasing power (centric–acentric) 0.58–0.75 0.42–0.50 0.33–0.34 Rcullis** 0.69 0.73 0.80 Mean FOM†† 0.46 Refinement Resolution, Å 40–1.65 Reflections, n 62,587 ‡‡ Rcryst, % 21.3 ‡‡ Rfree, % 24.1 Protein atoms, n 2797 Water atoms, n 407 rmsd bonds, Å 0.005 rmsd angles, ° 1.2 rmsd improper angles, ° 0.83 EMTS, sodium ethylmecurithiosalicylate. *Resolution range of the highest shell is listed in parentheses. †Completeness in the highest-resolution shell is listed in parentheses. ‡ Rsym ϭ͚͉Iobs Ϫ͗I͉͚͘͞Iobs, where Iobs is the observed intensity of reflection. Rsym in the highest-resolution shell is listed in parentheses. §I͞(I) in the highest-resolution shell is listed in parentheses. ¶ Riso ϭ͚͉Fder Ϫ Fnat͉͚͞Fnat, where Fnat and Fder are the native and derivative structure factor amplitude, respectively. ϭ ͚ 2 ͚͞ Ϫ 2 1/2 Phasing power ( FH (FPH(obs) FPH(calc)) ) , where FH represents the calculated heavy atom structure factor amplitude. **Rcullis ϭ͚ʈFPH Ϯ FP͉ Ϫ FH͉͚͉͞FPH Ϯ FP͉. †† Mean figure of merit (FOM) ϭ͗Fbest͞F͘. ‡‡ Rcryst,free ϭ͚͉Fobs Ϫ Fcalc͉͚͞Fobs, where the crystallographic R factor is calculated including and excluding refinement reflections. In each refinement, free reflections consist of 5% of the total number of reflections. multiple isomorphous replacement phases were carried out by and by analytical ultracentrifugation (Optima XL-1, Beckman using the program SOLVE (20).
Details
-
File Typepdf
-
Upload Time-
-
Content LanguagesEnglish
-
Upload UserAnonymous/Not logged-in
-
File Pages6 Page
-
File Size-