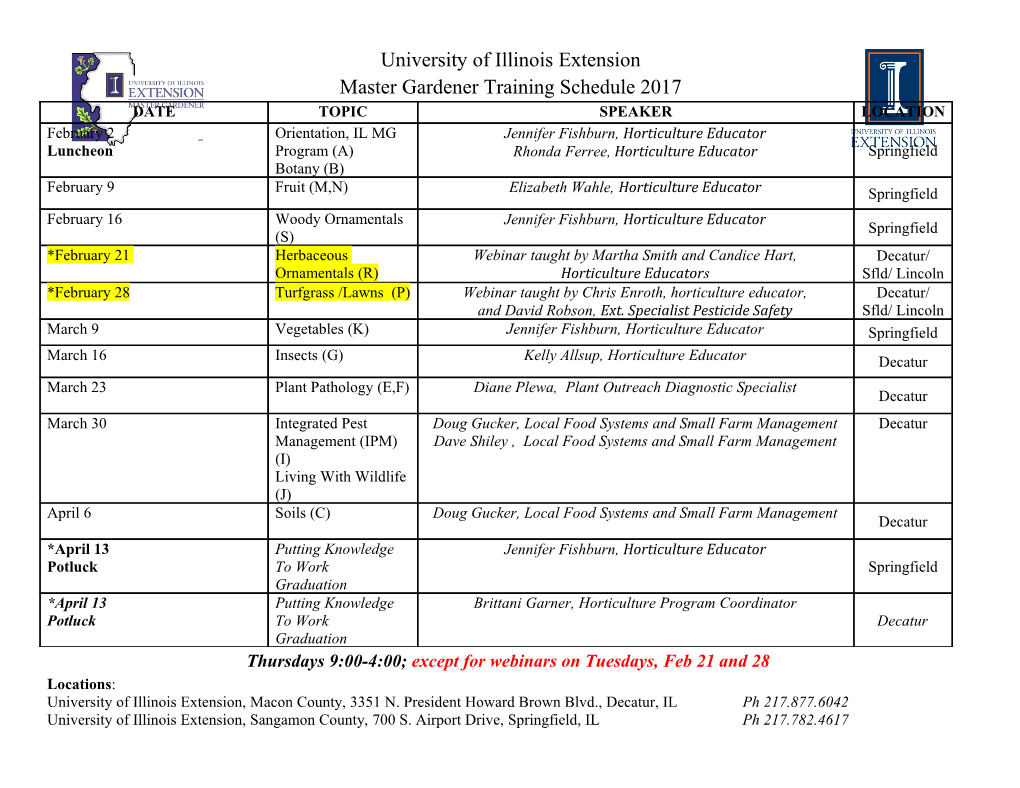
Copyright by John William Steele 2020 The Dissertation Committee for John William Steele Certifies that this is the approved version of the following dissertation: Mechanisms of Impaired Mitochondrial One-carbon Metabolism and Drug Exposures to Valproic Acid or Dolutegravir in Neural Tube Defects Committee: Richard H. Finnell, Co-Supervisor Dean R. Appling, Co-Supervisor Seema Agarwala John B. Wallingford Steven S. Gross Mechanisms of Impaired Mitochondrial One-carbon Metabolism and Drug Exposures to Valproic Acid or Dolutegravir in Neural Tube Defects by John William Steele Dissertation Presented to the Faculty of the Graduate School of The University of Texas at Austin in Partial Fulfillment of the Requirements for the Degree of Doctor of Philosophy The University of Texas at Austin May 2020 Dedication This dissertation is dedicated to my loving parents, James and Irene Steele. My work would not have been possible without their unfailing support and encouragement. Acknowledgements First, I must thank my mentor, Dr. Richard Finnell, who supported me even in the lowest and most challenging times of my graduate career. I would also like to thank Drs. Bogdan Wlodarczyk and Robert Cabrera, who have been my close mentors and shaped my understanding of science and my scientific approach. Dr. Linda Lin not only assisted me on most of my mouse experiments, but she has also been one of my dearest friends for the last six years. Nellie Chen performed thousands of genotyping reactions contributing to this work and has been the best undergraduate assistant for which a graduate student could ever hope. Xuanye Cao assisted with data analysis on RNA-seq experiments. Drs. Renu Pandey and Stefano Tiziani performed the LC-MS on VPA-treated embryos at Dell Pediatric Research Institute. Gabriel Tukeman assisted with the DTG cell culture experiments, and Dr. Robert Cabrera assisted with flow cytometry. Xue Gu, Jen Gilmore, Neeraj Patel, and Laura Guillen provided administrative support for my projects and made sure I always got paid. I also want to sincerely thank the following current or former members of the Finnell Lab who either assisted directly on my projects or otherwise contributed positively to my graduate experience: Sung-Eun Kim, Tian Tian, Yunping Lei, Hui Wei, Yael Pomerantz, Xiao Han, Jimi Kim, Amanda Vaughn, Manami Toriyama, and Rachel Tittle. I want to thank Sharon Bayliss, John Bayliss, and Dr. Tim George, who contributed significantly to my first publication as a graduate student, and I want to thank Baylor College of Medicine for hosting my graduate research for the last three years. Finally, I would like to thank my committee members, Drs. Dean Appling, Steven Gross, Seema Agarwala, and John Wallingford for their considerate advice and guidance. v Abstract Mechanisms of Impaired Mitochondrial One-carbon Metabolism and Drug Exposures to Valproic Acid or Dolutegravir in Neural Tube Defects John William Steele, Ph.D. The University of Texas at Austin, 2020 Supervisors: Richard H. Finnell and Dean R. Appling Neural tube defects (NTDs) are among the most severe and prevalent human congenital malformations. Their etiology is complex and multifactorial, influenced by dynamically interacting genetic and environmental factors. It is well known that maternal dietary folate status is the greatest modifying factor associated with risk for NTD-affected pregnancies, and that dietary fortification of folic acid (FA) can prevent a significant proportion of NTDs. However, many NTDs have proven to be FA-resistant, presenting a need to understand mechanisms underlying these FA-resistant defects and develop novel intervention strategies targeting this population. A class of FA-resistant NTD mouse models have been developed by inactivating genes associated with mitochondrial one- carbon metabolism (mOCM). Thus, Part One of this work sought to elucidate mechanisms by which impaired mOCM results in FA-resistant NTDs. By crossing mice heterozygous for loss of Slc25a32, a gene coding for the mitochondrial folate transporter, with mice heterozygous for the Crooked Tail (Cd) allele of Lrp6, a gene coding for a Wnt co-receptor, it was discovered that a proportion of resulting co-heterozygous offspring presented with vi NTDs, suggesting a novel digenic interaction between Lrp6 and mOCM. Further experiments demonstrated that Lrp6 regulates expression of mOCM genes in mouse embryos, while CHO cells lacking Slc25a32 demonstrated impaired Wnt signaling rescued by the one-carbon donor, glycine. Building on those data, it was discovered that maternal glycine supplementation could prevent NTDs in Slc25a32 null embryos, and that glycine or serine supplementation may reduce NTDs in Lrp6 Cd mice. Part One experiments also demonstrated that embryonic stem cells lacking Mthfd1l, another mOCM gene, have proliferation defects and are sensitive to hypoxia. Environmental exposure to certain pharmaceutical compounds also increases risk for NTDs. Part Two of this work sought to identify mechanisms of NTD pathology associated with two common pharmaceuticals, the anticonvulsant, valproic acid (VPA), and the HIV integrase inhibitor, dolutegravir (DTG). Untargeted metabolic profiling was performed on VPA-treated mouse embryos, and predictive biomarkers of VPA sensitivity were identified by comparing VPA-affected and unaffected embryos. Other experiments identified a novel, calcium-enhanced interaction between DTG, folate, and folate receptor, suggesting a plausible mechanism by which DTG may enhance NTD risk. vii Table of Contents List of Tables ................................................................................................................... xiii List of Figures ....................................................................................................................xv Chapter 1: Introduction ........................................................................................................1 1.1 Neural Tube Defects .............................................................................................1 1.1.1 Embryology of Neural Tube Defects .....................................................1 1.1.2 Etiology of Neural Tube Defects ...........................................................4 1.1.3 Prevention of Neural Tube Defects .......................................................6 1.2 One-carbon Metabolism .......................................................................................8 1.2.1 Folate .....................................................................................................9 1.2.2 Folate Transport ...................................................................................12 1.2.2.1 Folate Receptors.......................................................................12 1.2.2.2 Reduced Folate Carrier (SLC19A1) ........................................13 1.2.2.3 Proton Coupled Folate Transporter (SLC46A1) ......................14 1.2.2.4 Mitochondrial Folate Transporter (SLC25A32) ......................15 1.2.3 Compartmentalization of One-carbon Metabolism .............................16 1.2.3.1 Mitochondrial One-carbon Metabolism ...................................19 1.2.3.2 Cytosolic One-carbon Metabolism ..........................................22 1.2.3.3 The Methionine Cycle..............................................................23 1.3 Pharmaceutical Exposures and Neural Tube Defects .........................................24 viii PART ONE: MECHANISMS OF IMPAIRED MITOCHONDRIAL ONE-CARBON METABOLISM IN NEURAL TUBE DEFECTS..................................................................28 Chapter 2: Mechanistic Interactions between Lrp6 and Mitochondrial One-carbon Metabolism in Murine Neural Tube Defects ...............................................................29 2.1 Introduction .........................................................................................................29 2.2 Materials and Methods........................................................................................32 2.2.1 Mouse Work ........................................................................................32 2.2.1.1 General .....................................................................................32 2.2.1.2 Genotyping ...............................................................................32 2.2.1.3 Glycine, Serine, and Formate Supplementation Experiments ...................................................................................33 2.2.2 Isolation and Culture of Mouse Embryonic Fibroblasts ......................33 2.2.3 CHO and glyB Cell Culture .................................................................34 2.2.4 TCF/LEF Luciferase Assay .................................................................34 2.2.5 Quantification of Serum Folate............................................................36 2.2.6 RNA Extraction, qPCR, and RNA-seq ................................................37 2.2.6.1 RNA Extraction and Purification from Embryos and CHO Cells ...............................................................................................37 2.2.6.2 cDNA Synthesis and qPCR .....................................................37 2.2.6.3 RNA-seq ..................................................................................38 2.2.7 Statistics ...............................................................................................38 2.3 Results .................................................................................................................39
Details
-
File Typepdf
-
Upload Time-
-
Content LanguagesEnglish
-
Upload UserAnonymous/Not logged-in
-
File Pages207 Page
-
File Size-