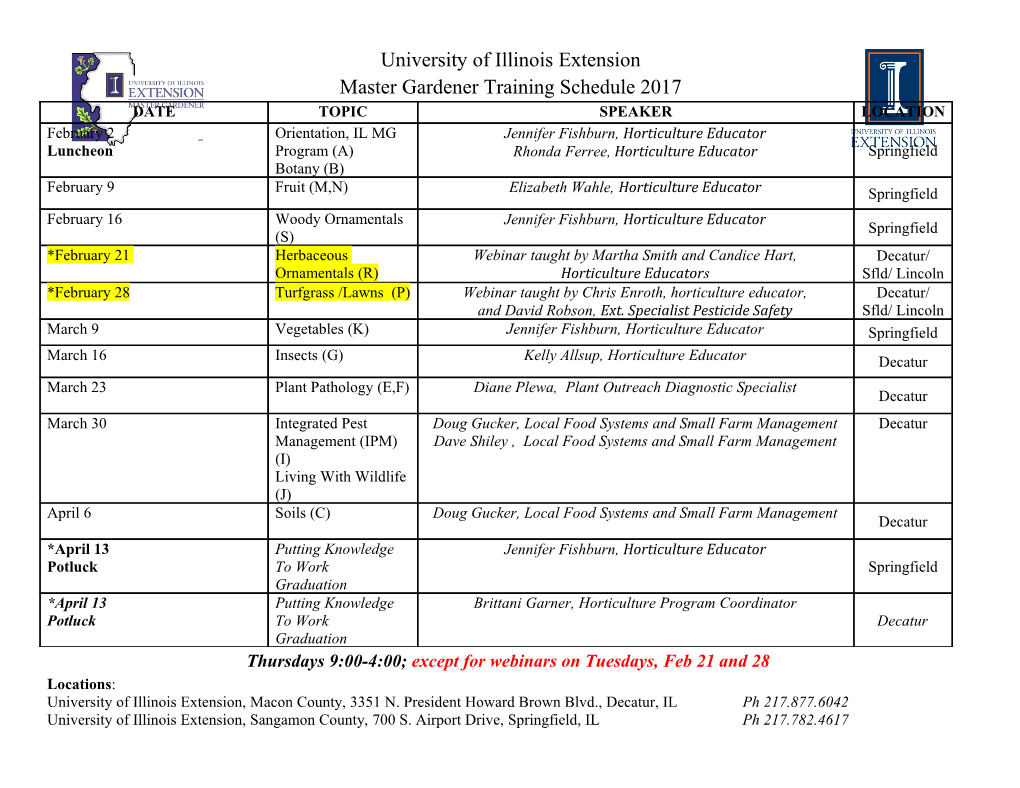
4 Amorphous silicon waveguides for microphotonics ½ Amorphous silicon a-Si was made by ion irradiation of crystalline silicon with 1¢10 ¾ Xe ions cm at 77 K in the 1–4 MeV energy range. Thermal relaxation of the amor- phous network at 500 Æ C for 1 hr leads to an amorphous layer with a refractive index of Ò Ò = 3.73, significantly higher than that of crystalline silicon ( =3.45at =1.55 m). a-Si can thus serve as a waveguide core in Si based optical waveguides. Channel wave- guides were made by anisotropic etching of a 1.5 m silicon-on-insulator structure that was partly amorphized. Transmission measurements of these waveguides as function of the amorphous silicon length show that the a-Si part of the waveguides exhibit a modal ½ ½ ½ propagation loss of 70 cm (0.03 dB m ) and a bulk propagation loss of 115 cm ½ (0.05 dB m ). Losses due to sidewall roughness are estimated, and are negligible com- pared to the modal loss. we conclude that a-Si waveguides are ideal for use in Si-based microphotonics applications on a 100 m length scale. 46 CHAPTER 4 4.1 Introduction Silicon is the most widely used semiconductor material in today’s electronic devices, be- cause of its excellent electronic properties. Single crystalline Si of high quality is available and the processing and microfabrication of Si are well known. Since silicon is transpar- ent in the near infrared, Si is also an ideal optical material. Si-based waveguides may serve as optical interconnects on Si integrated circuits, or to distribute optical clock sig- nals on a microprocessor. In such cases, using Si provides the advantage of integration with existing electronic circuits. So far optical waveguide technology in silicon is not well developed. Fabrication of waveguides in Si requires a core with a higher refractive index than that of crystalline Si (c-Si). Amorphous Si (a-Si) is an interesting candidate as a core material. While the density of pure a-Si is 1.8% lower than that of c-Si [72], the refractive index of pure a-Si, at near-infrared wavelengths, is higher than that of c-Si [60, 73–75]. Amorphous silicon can thus be used as a waveguide core material on crystalline Si. As pure a-Si contains a large density of point defects and dangling bonds, the optical absorption at near-infrared wavelengths can be substantial. These point defects can be passivated to some extent by incorporation of hydrogen. In fact, hydrogenated a-Si made by deposition shows excellent electrical quality, and relatively low optical absorption [76]. Amorphous silicon has already received a lot of interest both from a fundamental point of view and because of its possible use in solar cells and optoelectronic devices. Hydrogenated a-Si films can be deposited using a number of different techniques, includ- ing plasma enhanced chemical vapor deposition (CVD), RF sputtering and hot-filament CVD. The hydrogen content, void density, structural properties as well as optical and electronic properties have been studied intensively and depend critically on the exact pro- cessing conditions. In contrast, a-Si made by ion irradiation of crystalline silicon has reproducible properties that are insensitive to the preparation details, while voids seem to be absent in this material [77]. Heat treatment of pure a-Si made by ion irradiation induces structural relaxation [78] and reduces the defect density, which leads to an improvement in the optical and electronic properties. Overall, it seems that hydrogenated a-Si has better transparency in the near-infrared than pure a-Si. However, pure a-Si can be made more easily in a Si processing sequence, as it requires a single ion implantation step. Further- more, pure a-Si has larger thermal stability then hydrogenated a-Si. Given these pro’s and con’s of pure a-Si, it seems interesting to study its applicability in microphotonic inte- grated circuits. In this chapter we study the properties of amorphous silicon waveguides made using silicon-on-insulator (SOI) substrates and discuss the feasibility of a-Si wave- guides for silicon microphotonic channel waveguides and optical interconnects, and as a guiding layer in two-dimensional photonic crystals. 4.2 Properties of amorphous silicon To study the amorphization and structural relaxation of a-Si, (100) orienteded c-Si sam- ples were mounted on a copper block that was kept at liquid nitrogen temperature. Sam- ½ ¾ ples were irradiated with 1 ¢10 Xe ions cm in the 1–4 MeV energy range to form amorphous layers of different thickness. All irradiations were done with the ion beam in- cident under an angle of 7Æ with the surface normal to avoid ion channeling effects along AMORPHOUS SILICON WAVEGUIDES FOR MICROPHOTONICS 47 Figure 4.1: RBS channeling spectra of amorphous silicon made by ion irradiation of crys- ½ ¾ talline Si with 1.0¢10 Xe ions cm . The Xe ion energy was 1 MeV. Spectra are shown for the as implanted sample and for a sample annealed for 1 hr at 500 Æ C. For comparison a channeling spectrum of crystalline Si and a random Si spectrum are shown as well. The surface channel of Xe is indicated in the figure and the Gaussian distribution of Xe ions is clearly visible. the (100) crystal direction. After irradiation the amorphous silicon was heat treated at Æ 500 C for 1 hr in a vacuum tube furnace (base pressure 10 mbar). The amorphous layer thickness was measured by Rutherford backscattering spectrometry (RBS) channel- Æ ing measurements using 2 MeV He· ions at a scattering angle of 165 . Variable angle spectroscopic ellipsometry in the 300–1700 nm wavelength was used to determine the refractive index of a-Si and to obtain independent information on the amorphous layer thickness. The RBS channeling measurements for 1 MeV Xe irradiation are shown in Fig. 4.1. The data for ion irradiated samples are compared to channeling and random spectra of unirradiated crystalline Si. As can be seen the Si signal follows the random height start- ing from the Si surface channel around channel 280, up to a certain depth in the sample for both the as-implanted and the sample annealed at 500 Æ C for 1 hr. Annealing leads to the removal of point defects and amorphous pockets in the c-Si near the a-Si/c-Si interface, which sharpens the interface. In addition, solid phase epitaxial crystallization takes place at a growth rate of 0.083 A/s˚ at 500 Æ C [79, 80] leading to a shift of the interface. This explains the difference in channeling spectra between the as-implanted and annealed sam- ples. The Gaussian shaped profile around channel 390 is due to the implanted Xe ions. The peak of the implanted Xe distribution is roughly at the center of the a-Si thickness, and the Xe peak concentration is 0.08 at.%. From similar channeling measurements and 48 CHAPTER 4 Figure 4.2: Real (n) and imaginary (k) part of the index of refraction for relaxed amorphous silicon (drawn line) as function of wavelength, obtained from spectroscopic ellipsometry measurements. The index is compared to literature values for crystalline silicon (dashed lines) [81]. In the near-infrared part of the spectrum ( 1100 nm) the refractive index of amorphous is typically 0.3 higher than that of crystalline silicon, while the absorption is small. ½ ¾ annealing studies we conclude that all samples irradiated with 1 ¢10 Xe ions cm in the energy range 1–4 MeV, became amorphous up to the surface. The amorphous silicon thickness after annealing ranged from 0.6 m for 1 MeV irradiation to 2.0 mfor4MeV irradiation. Figure 4.2 shows the measured complex refractive index of amorphous silicon, made by 1 MeV Xe irradiation, after thermal relaxation (drawn line) compared to literature values for crystalline silicon (dashed line). After relaxation the ellipsometry data for wavelengths longer than 1.2 m can be described with a lossless layer with a refractive index 0.3 higher than that of crystalline silicon. To obtain the layer thickness accurately the ellipsometry data was first fitted to a model assuming a transparent layer in the range £ 1200 nm . The refractive index was then obtained for the entire wavelength range by point by point fitting of the complex refractive index, while keeping the layer thickness fixed. By comparing data for samples made using different Xe ion energies it was found that the optical properties are independent of layer thickness. Also, samples annealed for Æ 2 hrs at 500 Æ C or for 1 hr at 550 C gave the same results. The density of pure amorphous silicon is lower than that of crystalline silicon [72] and thus a density difference cannot ex- plain the higher refractive index. Hence the high refractive index at infrared wavelengths £ To fit the ellipsometry data correctly, a 2.41 nm thick native oxide layer on top of the amorphous silicon was used in the model. AMORPHOUS SILICON WAVEGUIDES FOR MICROPHOTONICS 49 must be attributed to a difference in the electronic bandstructure. Such a difference is apparent from the data near the direct band-to-band transitions in the visible part of the spectrum as observed in Fig. 4.2. Ellipsometry as performed here is not sensitive to very small optical absorption. Given the experimental error on the ellipsometry data, an upper ½ limit of the loss coefficient of amorphous silicon at 1.5 m is found: 100 cm . 4.3 Amorphous silicon waveguides To further investigate the optical losses of a-Si, commercially available silicon-on-insula- ª tor (SOI) substrates with a 1.5 m thick (100) oriented p-type (14–22 cm) Si layer on a ½ ¢ 3.0 mthickSiO¾ layer grown by wet thermal oxidation were ion irradiated with 1 10 ¾ 2 MeV Xe ions cm at T = 77 K.
Details
-
File Typepdf
-
Upload Time-
-
Content LanguagesEnglish
-
Upload UserAnonymous/Not logged-in
-
File Pages10 Page
-
File Size-