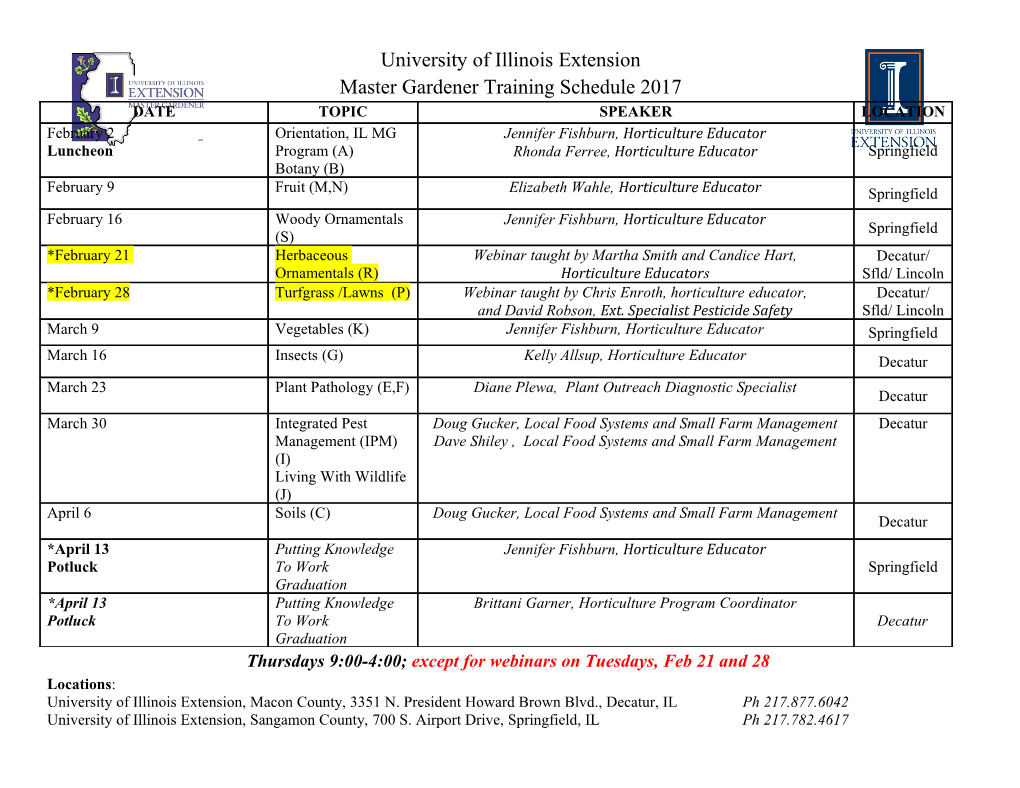
Development of Integrated Imaging Techniques for Investigating Biomarkers in Glioblastoma HIVE HEISOOG KIM B.S., Nuclear Engineering (2004) Seoul National University M.D. Certificate, Graduate Education in Medical Sciences (2011) Harvard-MIT Division of Health Sciences and Technology SUBMITTED TO THE DEPARTMENT OF NUCLEAR SCIENCE AND ENGINEERING IN PARTIAL FULFILLMENT OF THE REQUIREMENTS FOR THE DEGREE OF DOCTOR OF PHILOSOPHY IN NUCLEAR SCIENCE AND ENGINEERING AT THE MASSACHUSETTS INSTITUTE OF TECHNOLOGY SEPTEMBER 2011 @ 2011 Massachusetts Institute of Technology All rights reserved Signature of Author: Heisoog Kim Department of Nuclear Science and Engineering A.. ust 8"', 2011 Certified by Bruce R. n, Professor of Health Science and Technology/Nuclear Engineering All/7 Thesis Supervisor Certified by A. Gregory Sorensen, Professor at Harvard Medical School, Thesis Supervisor Reviewed by Elfar Adalsteinsson, Associate professor of Health Science and Technology, Thesis Reader Accepted by Mujid S. Kazimi, TEPCO P fess f Nuclear Engineering Chair, Department Committee on Graduate Students Table of Contents Abstract 5 Acknowledgements 7 List of Figures 9 List of Tables 11 1. Introduction 15 1.1. Significance of Developing Biomarkers 15 2. Cancer Physiology 19 2.1. Glioblastoma 19 2.2. Vascular Structure and Function in Brain Tumors 20 2.3. Angiogenesis in Tumor 22 2.4. Oxidative Metabolism in Tumors 23 2.5. Hypoxia in Tumors 26 3. Treatment Philosophies 33 3.1. Surgery 33 3.2. Radiotherapy 33 3.3. Chemotherapy 35 3.4. Antiangiogenic Therapy 37 4. Basics of Advanced Imaging Techniques 41 4.1. Magnetic Resonance Imaging Techniques 41 Simultaneous Blood Oxygenation Level Dependent (BOLD) and Arterial Spin Labeling (ASL) Imaging 42 Proton Magnetic Resonance Spectroscopy ('H MRS) Imaging 44 4.2. Positron Emission Tomography (PET) Imaging Technique 46 18F-FMISO PET Imaging 47 3 5. Angiogenesis Biomarker: Serial MR Spectroscopy Revealing an Antitumor Effect of Cediranib in Glioblastoma 55 5.1.Introduction 55 5.2. Methods 57 5.3. Results 61 5.4. Discussion 68 5.5. Appendix 75 6. Oxidative Metabolism Biomarker: A Significant Increase in Oxygen Consumption Rate in Hyperoxia in Glioblastoma 85 6.1. Introduction 85 6.2. Methods 88 6.3. Results 91 6.4. Discussion 99 7. Hypoxia Biomarker: A Simultaneous Measurement of Relative CMRO 2with MRI and '8F-MISO Uptake with PET in Glioblastoma 107 7.1.Introduction 107 7.2. Methods 110 7.3. Results 113 7.4.Discussion 116 8. Conclusion 121 8.1. Current state of Integrated Imaging Techniques for Biomarker Identification 121 8.2. Novel Methodologies 122 8.3. Future Work and Potential Applications 123 4 Development of Integrated Imaging Techniques for Investigating Biomarkers in Glioblastoma By HEISOOG KIM Abstract Cancer is a diverse disease with many manifestations. Various imaging modalities including magnetic resonance imaging (MRI) and positron emission tomography (PET) have been used to study human cancer. In this study, we developed integrated imaging techniques using advanced MR and PET to investigate potential biomarkers in glioblastoma (GBM). First, we applied proton magnetic resonance spectroscopy to assess the therapeutic effects of the new antiangiogenic drug (cedianib) on GBM. By evaluating changes in the levels of metabolites predominant in GBM during the treatment, we observed an antitumor response in GBM after one month. Notably, the index of the ratio of primary metabolites, NAA/Cho, in tumor strongly predicted 6-month overall survival; these data therefore suggest that NAA/Cho is the MRS- detectable biomarker that relates to tumor angiogenesis. Second, a simultaneous BOLD-ASL technique was investigated to measure the relative cerebral metabolic rate of oxygen (CMRO2) in hyperoxia (i.e., M CM 0 ) in GBM. Renewed interest in tumor metabolism, particularly in GBM, has recently prompted a re-examination of the Warburg effect. Our data have revealed that oxygen-induced CMRO 2 in tumor showed significant increase, supporting recent hypotheses on the preserved integrity of oxidative pathways in glycolytically active tumors. These data also propose a second remarkable biomarker for detecting tumor oxidative metabolic changes. Finally, and most importantly, we extended our earlier findings to explore the correlation between changes in oxidative metabolism and hypoxia level in GBM patients undergoing a multi-therapy 5 treatment protocol by acquiring simultaneous MRI-PET data using our novel dual-modality MR- PET imaging system. We observed an increase in relative CMRO2 in regions showing high-level uptake of our 1 8F-MISO probe in pre-treated tumor, and a subsequent large reduction in both values with tumor regression following treatment. The consistency between tumor oxidative physiology and hypoxia assessed by our novel integrated imaging approach will be a good biomarker for detecting oxidative changes with therapeutic effects in gliomas. Overall, our integrated imaging methods offer great potential to move from the preliminary biomarker stage to later stages with more clearly established utility. With further investigation, these imaging tools could contribute in significant ways to the ongoing effort to reduce morbidity and mortality in cancer. Thesis Supervisors: Bruce R. Rosen, M.D., Ph.D. Professor of Radiology, Harvard Medical School Professor of Health Science and Technology, Massachusetts Institute of Technology Director, A.A. Martinos Center, Massachusetts General Hospital A. Gregory Sorensen, M.D. Professor of Radiology, Harvard Medical School 6 Acknowledgements "This, too, shall pass away." IJk 614,1 ' q-t -11 jv I J7 %j7E I. -- - 4t 11 V !-qam I-J- TF 0 ±P31 i 3 =L-A] 4-k! "I-A147. vj : 1 A] M 7f4 i 371 i&EE- !a~i MIT 4V Harvard Medical School Oil Ai Ij Ai it 1 7P 1 %54 Y - "04~ 0-!-Qualifying Exam --- ]t 1 P'0101 ri 4, 1147o-8 01 -'F -& -- E]"r 2Ii±F WE ~ TA 4} RA 4-- 1M 4 - 1 42" 1 1,-' -O~IJ 10kg 1-jA1 ~- 14 -;'l 4 JK-7m! ~11 701rq 90, 0 1T4 4 -111 A]1 4 F± 2 w'"1 oi i4-K-:A 41-1 4 wA1 '4 - o -k 1C-- -X-- ;4 1 5 4 4a-U4 0,0 42d1' qar} I- -Vt17971 m -ig-L]?] 51!4PAq-qO~~ jj 4J ~ 1- rc I71~-%%7A {h ~ 1r- ~ ~- -6 014 2x:=- 01eg ~1= IJ~ 'IJA- tlj-3-qI 1 4~ Oi L= 7 ~~4}. 4 kI ll~ d 7A9 9 9~2,L' + , 'U± 9 2J }~~ 7 *Il, o 0V J Ai e1 1 - o}0 9 1 Yk7 }il l-4- Ol AJ A~l A] EOti.0'%f 1 rfl T31-.r}i A1401 %r1 2-4oj1-. 2Lzi4 ol7~ M01Jo o}'d? 171*i 71L avl 2 - 7l~~ One day in Summer 2011 HAEJIN in Boston 8 List of Tables Table 1 Stages of biom arker developm ent ................................................................................. 17 Table 2 Mean and standard deviation of NAA/norCre, Cho/norCre,and NAA/Cho on day -5 and day -1 for all the patients; their coefficients of variance and p values between two baselines; in three R O Is..........................................................................................................................64 Table 3 Area Under the ROC curve on early time points (i.e. 1 day, 28 day, 56 day) to determine the prediction of NAA/Cho to 6-month survival...............................................................67 Table 4 Heart rate (HR) and oxygen saturation level (i.e., saturation of peripheral oxygen, SPo2) at baseline and at oxygen for all healthy volunteers ......................................................... 92 Table 5 Heart rate (HR) and oxygen saturation level (i.e. saturation of peripheral oxygen, SPo2) at baseline and at oxygen for all nGBM patients.......................................................................92 Table S 1 Number of subjects included in the analysis at each time point ................................ 79 9 10 List of Figures Figure 1 A schematic view of the relationship between biomarkers and the new molecular entity, its target, the disease pathophysiological cascade, and their impact on stages of drug developm ent...........................................................................................................................17 Figure 2 Schematic representation of the differences between unicellular and multicellular organism s in energy m etabolism .................................................................................... 25 Figure 3 a. Chemical structure of '8F-MISO; b. mechanism of '8F-MISO cell trapping............48 Figure 4 Three regions of interest (ROIs) were defined on the corresponding Ti-weighted post- contrast images: (1) enhancing tumor (red voxels), (2) non-enhancing surrounding tumor - i.e. peritumoral tissue (blue voxels), and (3) normal tissue on the contralateral side of tumor (green voxles). To obtain an accurate assessment of tumor metabolism, the voxels in enhancing tumor were selected by avoiding areas of necrosis, hemorrhage, calcification, or cy sts. ...................................................................................................................................... 6 0 Figure 5 Serial TI post-contrast MR images and raw spectra in one representative voxel (blue- lined box) of enhancing tumor region in the time course of treatment. The spectra exhibit the dynamic changes of each metabolite's peak in the range of 0.5 - 4 ppm at every time point of treatm ent...................................................................................................................65 Figure 6 Averaged MRS changes over all eligible patients relative to pretreatment values (%)...66 Figure 7 the relative changes (%) in NAA/Cho separately grouped by the patients' overall survival (OS) periods based on six-month survival threshold at the early time points
Details
-
File Typepdf
-
Upload Time-
-
Content LanguagesEnglish
-
Upload UserAnonymous/Not logged-in
-
File Pages125 Page
-
File Size-