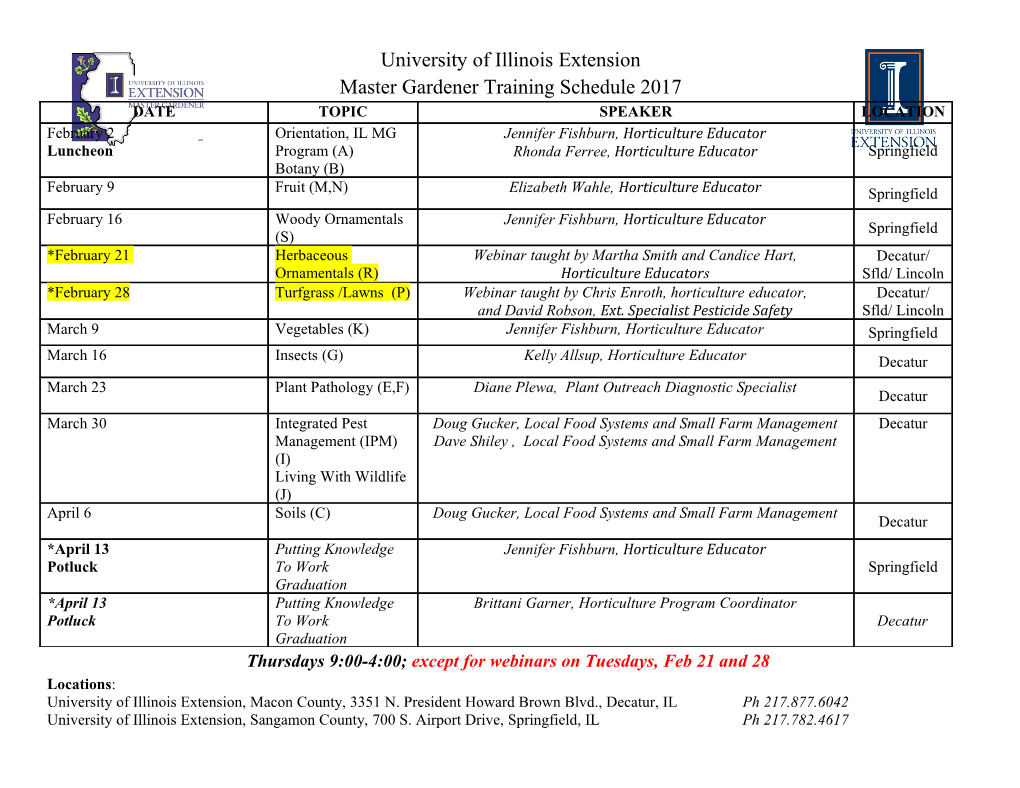
Downloaded from genesdev.cshlp.org on September 25, 2021 - Published by Cold Spring Harbor Laboratory Press The scalloped gene encodes a novel, evolutionarily conserved transcription factor required for sensory organ differentiation in Drosophila Shelagh Campbell/'^ Maneesha Inamdar,^ Veronica Rodrigues/ Vijay Raghavan,^ Michael Palazzolo/ and Arthur Chovnick^'^ ^Department of Molecular and Cell Biology, University of Connecticut, Storrs, Connecticut 06269-2131 USA; ^Molecular Biology Unit, Tata Institute of Fundamental Research, Homi Bhabha Road, Colaba, Bombay 400005, India; ^Department of Genetics, Washington University Medical School, St. Louis, Missouri 63110 USA The scalloped {sd) gene of Drosophila melanogaster was initially characterized by mutants affecting structures on the wing of the adult fly. The sequence of a cDNA clone of the gene reveals a predicted protein sequence homologous to that of a human transcriptional enhancer factor, TEF-1 (68% identity). The homology includes a sequence motif, the TEA domain, that was shown previously to be a DNA-binding domain of TEF-1. An sd enhancer trap strain expresses the reporter gene in a subset of neuroblasts in the central nervous system and in the peripheral sense organs of the embryo. The reporter gene is later expressed in specific regions of the imaginal discs, including regions of the wing disc destined to become structures defective in viable sd mutants. Later still, expression in the adult brain is restricted to subsets of cells, some in regions involved in the processing of gustatory information. These observations indicate that the sd gene encodes a transcription factor that functions in the regulation of cell-specific gene expression during Drosophila development, particularly in the differentiation of the nervous system. [Key Words: Drosophila-, scalloped genc; TEF-1\ Received December 5, 1991; revised version accepted January 3, 1992. One approach to identifying genes with roles in the de­ lido 1988). A cell-autonomous sd~^ function is required velopment and function of the nervous system is to char­ during larval development for normal wing development acterize mutants with phenotypic defects affecting the (Simpson et al. 1981). Histological analysis of a viable sd external sensory organs. Hypomorphic mutations in the mutant indicates elevated cell death in larval wing discs, scalloped [sd] gene of Drosophila melanogaster affect suggesting that the adult wing phenotype is the result of the morphology of the adult wing (Gruneberg 1929). This loss of precursor cells of the wing margin (James and phenotype involves the loss of sensory structures that Bryant 1981). Cell autonomy of the wing function may normally comprise the wing margin^ creating a gapped or mean that products of this gene act in the reception or scalloped appearance. The phenotype is similar to that of implementation of developmental signals. Lethal sd al­ mutant alleles of several genes with demonstrated roles leles that die either as early larvae or during pupal de­ in neural development: cut (Bodmer et al. 1987), Notch velopment have been characterized, and all but one of (Campos-Ortega and Knust 1990; Xu et al. 1990), and these fails to complement the wing phenotype in trans- Serrate (Fleming et al. 1990; Thomas et al. 1991). In ad­ heterozygote combinations with different viable sd alle­ dition to margin defects, sd mutants often develop ec­ les (Campbell et al. 1991). Recently, it has been found topic macrochaetae on the wing blade (Campbell et al. that viable sd mutants also show abnormal responses to 1991). This aspect of the sd mutant phenotype is similar gustatory stimuli (Anand et al. 1990). to that of other genes with regulatory functions in neural The sd gene was cloned by transposon tagging with a development: hairy (Rushlow et al. 1989), shaggy/zw-3 P[ry'^] insertion mutation and chromosome walking (Perrimon and Smouse 1989; Simpson and Carteret 1989) (Daniels et al. 1985; Campbell et al. 1991). Viable and and extramacrochaetae (Garcia Alonso and Garcia-Bel- lethal sd mutations were mapped within a ~14-kb geno­ mic region, and structurally related, developmentally regulated transcripts hybridizing to this region were ^Present address: Department of Biochemistry, University of California, San Francisco California 94143-0448 USA characterized (Campbell et al. 1991). cDNA clones rep­ ^Corresponding author. resenting three transcript classes were identified that are GENES & DEVELOPMENT 6:367-379 © 1992 by Cold Spring Harbor Laboratory Press ISSN 0890-9369/92 $3.00 367 Downloaded from genesdev.cshlp.org on September 25, 2021 - Published by Cold Spring Harbor Laboratory Press Campbell et al. presumed to arise by alternative RNA splicing of a —12- siae (Laloux et al. 1990), and the human transcription kb primary transcription unit. In this report we describe enhancer factor, TEF-1 (Xiao et al. 1991). Among the four the sequence and exon structure of a cDNA clone repre­ identified members of this new class of genes, the TEA senting one of these sd transcripts. The potential trans­ domain of sd is most closely related to that of the human lation product of this sd cDNA clone includes a highly gene TEF-1 (70/72 residues, 98% identical). In compari­ conserved sequence motif described recently as the son, the TEC-1 and abaA proteins are only 44% and 65% "TEA domain/' proposed to be the signature of a new identical to TEF-1 over the TEA domain, respectively family of DNA-binding proteins (Biirglin 1991). (Biirglin 1991). The DNA-binding domain of the TEF-1 We have found an sd enhancer trap strain that has a protein corresponds to the conserved TEA domain (Xiao p-galactosidase reporter gene inserted within an intron etal. 1991). of the sd gene. The reporter gene is expressed in cells of Sequence conservation between sd and TEF-1 extends the central nervous system (CNS) and peripheral nervous beyond the TEA domain and includes almost the entire system (PNS) in a dynamic and complex pattern. These predicted protein sequence (Fig. 2). Allowing for gaps to observations suggest that sd participates in a regulatory maximize the alignment between TEF-1 and scalloped network controlling the differentiation of the nervous predicted protein sequences, we observe 68% identical system. We discuss these findings and directions for fu­ amino acids and 81% similar amino acids over the entire ture studies. aligned sequence. The predicted sd protein includes an additional 51 amino acids at its 5' end relative to the TEF-1 protein but is predicted to end at an identical car- Results boxy 1-terminal residue (Fig. 2). Both TEF-1 and the sd- predicted protein sequence have serine-rich amino-ter- An sd cDNA clone encodes a homolog of the human minal regions, followed by the basic TEA domain. A pro­ transcription enhancer factor gene, TEF-1 posed "finger" motif at the carboxyl terminus of the The £21 cDNA clone sequenced in this study was recov­ TEF-1 gene is only partially conserved in the sd sequence ered from an embryonic cDNA library probed with ge­ (see legend to Fig. 2). nomic DNA from a region altered in several lethal sd alleles (Brown and Kafatos 1988; Campbell et al. 1991). Structure of the sd transcription unit The E2I clone is 2938 bp in length and hybridizes to genomic DNA fragments extending across a ~12-kb ge­ To determine the structure of the transcript represented nomic region that encompasses the genomic interval af­ by the E21 clone, DNA clones of sd genomic restriction fected by DNA lesions in a number of different viable fragments that hybridize to it were sequenced and the and lethal sd mutations. The sequence of the E21 cDNA genomic and cDNA sequences were aligned to identify clone is presented in Figure 1. A large open reading frame the boundaries of exon and intron domains. The E21 (ORF) of 1323 bp is found in this sequence, and a trans­ clone has 12 exons whose positions relative to the geno­ lation of the ORF is shown in Figure 1. We have used mic map are shown in Figure 3 (see also Fig. 1 for the PCR amplification of genomic DNA to demonstrate that positions of intron/exon boundaries within the cDNA one of the lethal sd mutants, l(l)sd'^^'^, has a ~100-bp sequence). The sequence data agree with previous esti­ deletion that would truncate the E21 clone within the mates based on genomic hybridization analysis in show­ ORF described in Figure 1 (see Fig. 3, below, for the ge­ ing that the primary sd transcription unit extends —12 nomic location of this deletion). The l(l)sd'^''^ allele is kb on the genomic map, as shown in Figure 3. Conven­ one of two larval-lethal sd alleles, suggesting that the tional splice site usage (Mount 1982) was observed at translation product encoded by the E21 cDNA clone is the intron/exon boundaries of the E21 clone (data not required for an early essential sd function. shown). The 440-amino-acid protein sequence encoded by the The 5' end of the E21 clone begins at the same geno­ ORF of the E21 clone was compared with sequences in mic position as another sd cDNA clone, D16, and both the GenBank data base, and a single significant match have a G residue at their 5' end that is not genomically was found that involved a stretch of 46 amino acids in a encoded. This feature has been noted for previously char­ gene cloned from Aspergillus nidulans called abacus A acterized complete cDNA clones isolated from this li­ [abaA; Mirabito et al. 1989). This sequence is part of an brary and is thought to be a cloning artifact that occurs evolutionarily conserved motif, the TEA domain, that when the reverse transcriptase attempts to copy the has been found recently in genes from two additional 7-methylguanosine cap of the mRNA during synthesis of organisms, the TEC-1 gene from Saccharomyces cerevi- the cDNA library (Brown et al.
Details
-
File Typepdf
-
Upload Time-
-
Content LanguagesEnglish
-
Upload UserAnonymous/Not logged-in
-
File Pages14 Page
-
File Size-