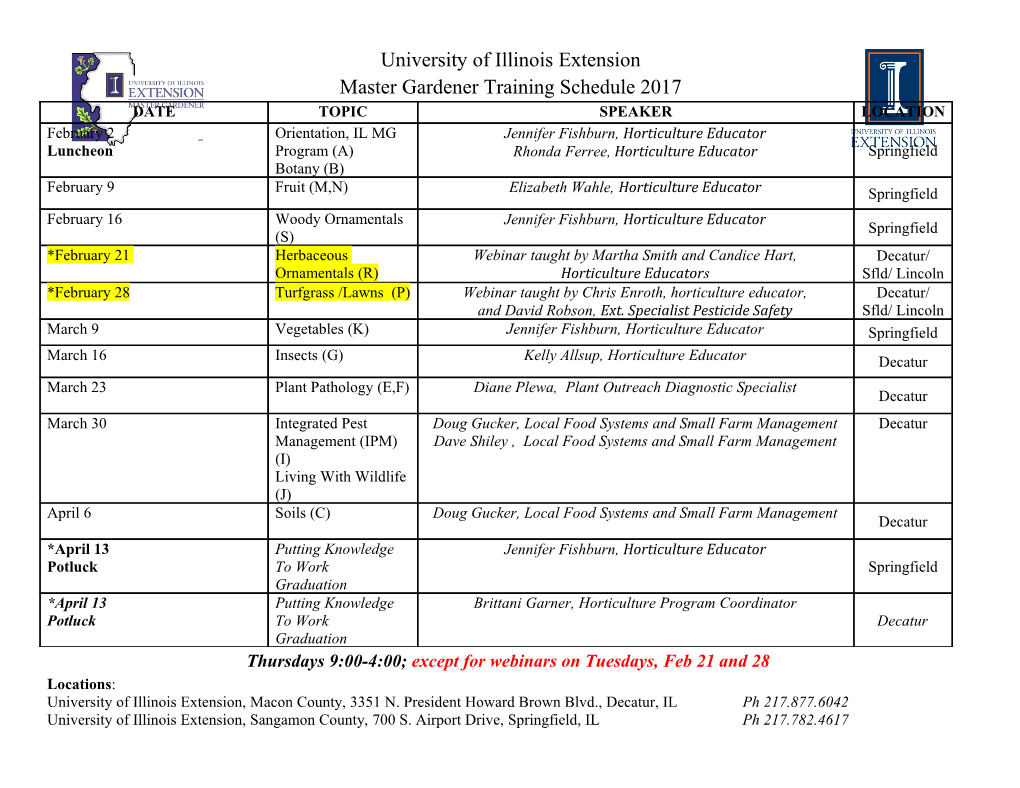
Vegetative Growth 19 and Organogenesis lthough embryogenesis and seedling establishment play criti- A cal roles in establishing the basic polarity and growth axes of the plant, many other aspects of plant form reflect developmental processes that occur after seedling establishment. For most plants, shoot architecture depends critically on the regulated production of determinate lateral organs, such as leaves, as well as the regulated formation and outgrowth of indeterminate branch systems. Root systems, though typically hidden from view, have comparable levels of complexity that result from the regulated formation and out- growth of indeterminate lateral roots (see Chapter 18). In addition, secondary growth is the defining feature of the vegetative growth of woody perennials, providing the structural support that enables trees to attain great heights. In this chapter we will consider the molecular mechanisms that underpin these growth patterns. Like embryogenesis, vegetative organogenesis and secondary growth rely on local differences in the interactions and regulatory feedback among hormones, which trigger complex programs of gene expres- sion that drive specific aspects of organ development. Leaf Development Morphologically, the leaf is the most variable of all the plant organs. The collective term for any type of leaf on a plant, including struc- tures that evolved from leaves, is phyllome. Phyllomes include the photosynthetic foliage leaves (what we usually mean by “leaves”), protective bud scales, bracts (leaves associated with inflorescences, or flowers), and floral organs. In angiosperms, the main part of the foliage leaf is expanded into a flattened structure, the blade, or lamina. The appearance of a flat lamina in seed plants in the middle to late Devonian was a key event in leaf evolution. A flat lamina maximizes light capture and also creates two distinct leaf domains: adaxial (upper surface) and abaxial (lower surface) (Figure 19.1). Several types of leaves have evolved based on their adaxial–abaxial leaf structure (see WEB TOPIC 19.1). © 2014 Sinauer Associates, Inc. This material cannot be copied, reproduced, manufactured or disseminated in any form without express written permission from the publisher. 554 Chapter 19 (A) Shoot structure and leaf polarity (B) Simple leaves Apical Apical meristem Blade Margin Midrib Vein Margin Midrib Petiole Sessile Node Distal (no petiole) Adaxial Stipule Sheath Petiole Proximal Axillary bud Abaxial Basal (C) Compound leaves Leaet Rachis Pinnately trifoliolate Palmate Paripinnate Bipinnate Tripinnate Figure 19.1 Overview of leaf structure. (A) Shoot struc- leaves. Variations in lower leaf structure include the pres- ture, showing three types of leaf polarity: adaxial–abaxial, ence or absence of stipules and petioles, and leaf sheaths. distal–proximal, and midrib–margin. (B) Examples of simple (C) Examples of compound leaves. In the majority of plants, the leaf blade is attached to the marginal expansion of leaf tissues, differentiation into the stem by a stalk called the petiole. However, some adaxial and abaxial domains, and morphogenesis along plants have sessile leaves, with the leaf blade attached the proximal-distal axis. Compound leaves are produced directly to the stem (see Figure 19.1B). In most monocots by variations on these developmental pathways. Finally, and certain eudicots the base of the leaf is expanded into we will discuss the gene networks and hormonal signals a sheath around the stem. Many eudicots have stipules, that control the development of the specialized cells of the small outgrowths of the leaf primordia, located on the epidermis and vascular tissue. Plantabaxial Physiology side of 6/E the leafTaiz/Zeiger base. Stipules protect the young Sinauerdeveloping Associates foliage leaves and are sites of auxin synthesis Morales Studio The Establishment of Leaf Polarity TZ6e_19.01during early leaf development. Date 09-10-14 Leaves may be simple or compound (see Figures 19.1B All leaves and modified leaves begin as small protuber- and C). A simple leaf has one blade, whereas a compound ances, called primordia, on the flanks of the shoot apical leaf has two or more blades, the leaflets, attached to a meristem (SAM) (see Chapter 17). All SAMs in higher common axis, or rachis. Some leaves, like the adult leaves plants share a common structure: a central, often dome- of some Acacia species, lack a blade and instead have a shaped domain surrounded by several emerging pri- flattened petiole simulating the blade, the phyllode. In mordia, which may be leaf primordia or, in the case of some plants the stems themselves are flattened like blades an inflorescence meristem, flower primordia. Cells in the and are called cladodes, as in the cactus Opuntia. apical meristem are considered undifferentiated and plu- We will begin our discussion of foliage leaf develop- ripotent. Nevertheless, as we saw in Chapter 17, the cells ment with the production of leaf primordia. Next we will of the SAM are organized into three more or less stable examine blade formation in simple leaves, which involves tissue layers—L1, L2, and L3—although some plants, © 2014 Sinauer Associates, Inc. This material cannot be copied, reproduced, manufactured or disseminated in any form without express written permission from the publisher. Vegetative Growth and Organogenesis 555 (A) (B) Figure 19.2 Longitudinal section of the Arabidopsis inflorescence meristem and dia- grams showing its functional organization. Flower (A) Light micrograph of the Arabidopsis inflo- L1 CZ L2 rescence meristem showing the localization PZ L3 I P of CLAVATA3 (CLV3 ) gene expression (brown Rib stain) (see Chapter 17). (B) Anatomical zona- zone tion of the inflorescence meristem showing the central zone (CZ), peripheral zone (PZ), flower primordium (P), flower primoridium initial (I), L1–L3 layers, flower, and rib zone. (C) Spatial variations in the rate of cell division (indicated by color bar), showing the highest rates in the (C) (D) flower primordia. (D) Proposed distribution of the three main hormones, auxin, cytokinin, and gibberellins, as well as sites of auxin transport, auxin depletion, and gibberellin (GA) degrada- tion. (From Besnard et al. 2011.) Cell cycle rate will discuss auxin canalization in more detail Low High later in this chapter.) Other hormones, such Auxin Auxin transport as cytokinin, gibberellins, and brassino- Cytokinin Auxin depletion steroids, also play critical roles in maintaining Gibberellin GA degradation SAM structure and activity. The distributions of auxin, cytokinin, and gibberellins in the SAM are shown in Figure 19.2D. such as maize (corn; Zea mays), lack L3. These tissue layers The initiation of leaf primordia was recently shown to can be further demarcated into three histological zones: be light-dependent in a manner that is independent of the central zone (CZ), peripheral zone (PZ), and rib zone photosynthesis; tomato and Arabidopsis apices cease pro- (RZ) (Figure 19.2A and B). ducing new leaf primordia when the plants are grown in To a large extent, positional information dictates the the dark. This cessation is correlated with decreased auxin fate of cells in the SAM. For example, the highest rates synthesis and loss of the polar localization of PIN1 in the of cell division in the inflorescence meristem of Arabi- SAM. However, because organ initiation in dark-grown dopsis are found in the primordium (P) and primordium apices can be restored only after application of both auxin initial (I), followed by the PZ and the CZ (Figure 19.2C). and cytokinin, it appears that cytokinin may be involved Position also determines the patterns of intracellular and in a light-dependent leaf initiation pathway. Phytochrome intercellular signaling. As we discussed in Chapter 17, B has been implicated as the photoreceptor involved in the the different histological zones exhibit distinctive pat- response to light (see Chapter 16), as it regulates auxin terns of gene expression that maintain the growing SAM synthesis and overall auxin levels in the plant. as a stable structure. In addition to hormonal signals, mechanical stress in the SAM has been shown to alter microtubule arrange- Hormonal signals play key roles ments as well as PIN1 distribution, which can affect leaf in regulating leaf primordia emergence Plant Physiology 6/E Taiz/Zeiger primordia initiation (see WEB TOPIC 19.2). SinauerAs we discussed Associates in Chapter 17, polar auxin transport in the INL1 HOUSE layer of the SAM is essential for leaf primordia emer- A signal from the SAM initiates TZ6e_19.02 Date 09-11-14 gence, and is responsible for leaf phyllotaxy (the pattern adaxial–abaxial polarity of leaf emergence from the stem; see Figure 17.32). When Since leaf primordia develop from a group of cells on the shoot apices are cultured in the presence of auxin trans- flank of the SAM, leaves possess inherent positional rela- port inhibitors they fail to form primordia, and application tionships with the SAM: the adaxial side of a leaf primor- of auxin to the SAM results in the induction of primordia dium is derived from cells adjacent to the SAM, while the at the application site. Auxin synthesis via the YUCCA abaxial side is derived from cells farther away. Microsurgi- pathway (see Chapter 15) generates auxin concentration cal studies in the 1950s demonstrated that some type of gradients which, in turn, regulate expression and asym- communication between the SAM and the leaf primor- metric distribution of PIN auxin efflux carriers to
Details
-
File Typepdf
-
Upload Time-
-
Content LanguagesEnglish
-
Upload UserAnonymous/Not logged-in
-
File Pages38 Page
-
File Size-