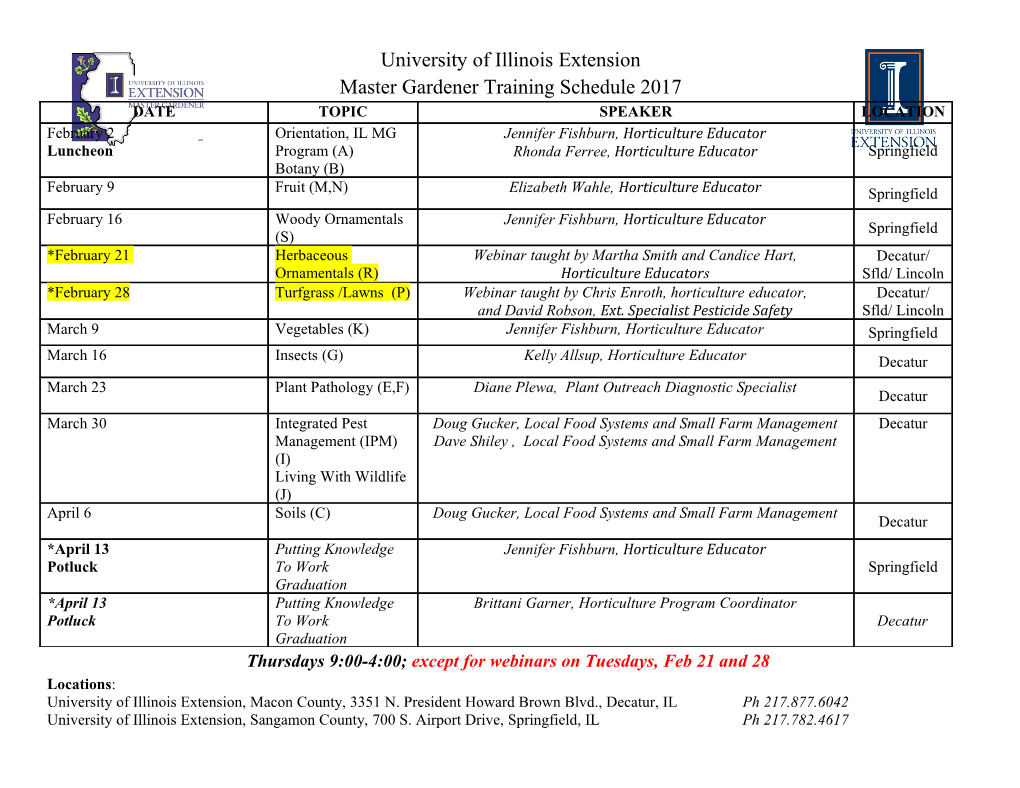
1 Title: Genomic adaptations in information processing underpin trophic strategy in a whole- 2 ecosystem nutrient enrichment experiment 3 4 Authors: Jordan G. Okie1,2, Amisha T. Poret-Peterson3, Zarraz M.P. Lee2, Alexander Richter4, Luis D. 5 Alcaraz5, Luis E. Eguiarte6, Janet L. Siefert7, Valeria Souza6, Chris L. Dupont4, and James J. Elser2,8 6 7 Affiliations: 8 1School of Earth and Space Exploration, Arizona State University, Arizona, USA. 9 2School of Life Sciences, Arizona State University, Arizona, USA. 10 3ARS Crops Pathology and Genetic Research Unit, USDA, California, USA. 11 4 J. Craig Venter Institute, California, USA. 12 5 Departamento de Biología Celular, Facultad de Ciencias, Universidad Nacional Autónoma de 13 México, Mexico. 14 6Departamento de Ecología Evolutiva, Instituto de Ecología, Universidad Nacional Autónoma de 15 México, Mexico. 16 7Department of Statistics, Rice University, Texas, USA. 17 age, University of Montana, Montana, USA. 18 Email addresses (in authorship order): [email protected], [email protected], 19 [email protected], [email protected], [email protected], [email protected], 20 [email protected], [email protected], [email protected], [email protected] 21 Running head: Genomics of r/K selection strategy 22 23 Keywords: Metagenomics, microbial communities, phosphorus fertilization, genome size, codon usage 24 bias, GC content, rRNA operon copy number, ecological stoichiometry, growth rate hypothesis, r/K 25 selection theory. 26 27 28 Corresponding author: Jordan G. Okie, School of Earth and Space Exploration, Arizona State 29 University, PO Box 871404, Tempe, AZ 85287, Phone: 505-366-1218; Fax: 480-965-8960; Email: 30 [email protected] 31 Data accessibility statement: Data has been deposited in appropriate public repositories and the DOI 32 made available at the end of the paper. 1 33 34 Abstract 35 Several universal genomic traits affect trade-offs in the capacity, cost, and efficiency of biochemical 36 information processing underpinning metabolism and reproduction. We analyzed their role in 37 mediating planktonic microbial community responses to nutrient enrichment in an oligotrophic, 38 phosphorus-deficient pond in Cuatro Ciénegas, Mexico—one of the first whole-ecosystem 39 experiments involving replicated metagenomic assessment. Mean bacterial genome size, GC content, 40 total number of tRNA genes, total number of rRNA genes, and codon usage bias in ribosomal 41 protein sequences were higher in the fertilized treatment, as predicted assuming oligotrophy favors 42 lower information-processing costs while copiotrophy favors higher processing rates. Contrasting 43 changes in trait variances also suggested differences between traits in mediating assembly under 44 copiotrophic versus oligotrophic conditions. Trade-offs in information-processing traits are 45 apparently sufficiently pronounced to play a role in community assembly as the major components 46 of metabolism—information, energy, and nutrient requirements—are fine-tuned to an organism’s 47 growth and trophic strategy. 48 49 2 50 Introduction 51 Traits influencing the informational underpinnings of metabolism may be crucial to performance 52 and community assembly but ecologists have largely focused on the proximal energetic and 53 stoichiometric features of metabolism (Leal et al., 2017; Sibly et al., 2012; Sterner and Elser, 2002). 54 Organisms must be able to store, copy, and translate the information contained in genetic material. 55 And, they must be able to adaptively update their transcriptome and proteome in response to altered 56 environmental conditions. To grow and reproduce rapidly, rates at every step of the metabolic 57 network must be sufficiently high such that no single step is unduly rate-limiting, including the 58 information processes that underpin biosynthesis and regulate metabolic networks. This necessary 59 integration of functions is a hallmark of all organisms. 60 The structure and size of the genome affect the rate, efficiency, and robustness of the 61 information processes supporting metabolism, growth, and reproduction (Appendix 1). There are 62 necessary tradeoffs in the costs and benefits of these features (Appendix 1; see also Smith, 1976), 63 which should consequently make individual organisms most competitive and better-suited for only 64 particular ranges of growth and trophic conditions (Roller and Schmidt, 2015). Organisms best 65 suited to compete in environments with abundant resources (copiotrophs) must have the capacity 66 for sufficiently high intracellular rates of information processing to support high rates of metabolism 67 and reproduction. However, maintaining the genomic and structural capacity for rapid growth is 68 costly, potentially placing such taxa at a disadvantage in stable, nutrient-poor environments where 69 growth rates are chronically slow (Giovannoni et al., 2014). Oligotrophic environments may thus 70 instead favor organisms (oligotrophs) that have information processing machinery that is less costly 71 to build, maintain, and operate, thereby increasing resource use efficiency and growth efficiency 72 (Koch, 2001; Roller and Schmidt, 2015). 3 73 Genomic traits affecting the rates and costs of biochemical information processing within 74 cells can thus influence the degree to which an organism is optimized for oligotrophy versus 75 copiotrophy. This oligotrophic-copiotrophic strategy continuum is reminiscent of the classic slow- 76 fast life history continuum (Stearns, 1992), classical r/K selection theory (MacArthur and Wilson, 77 2001; Pianka, 1972, 1970), and their subsequent developments dealing with the evolution of 78 interspecific variation in rates of resource use, mortality, growth, and reproduction (e.g., Dobson, 79 2012; Grime and Pierce, 2012; Krause et al., 2014; Sibly and Brown, 2007). In conjunction with 80 research on the role of functional traits and niches in shaping the assembly of communities (e.g., 81 Fukami et al., 2005; Litchman and Klausmeier, 2008; McGill et al., 2006; Okie and Brown, 2009; Roller 82 and Schmidt, 2015), this work suggests traits associated with biological rates and efficiencies of 83 resource use, growth, and reproduction play an important role in community assembly. It is unclear, 84 however, whether the traits specifically related to rates and costs of biochemical information 85 processing have sufficiently pronounced tradeoffs or physiological effects to play an important role 86 in the evolutionary ecology of organisms and assembly of communities, although there are some 87 promising indications (Dethlefsen, 2004; Roller et al., 2016). 88 Here, coupling metagenomic analysis with a trait-based framework that synthesizes theory 89 and hypotheses from genomics, ecology, and evolutionary cell biology, we investigate the role that 90 several universal genomic traits play in determining the response of a planktonic microbial 91 community to nutrient enrichment in a whole-ecosystem experiment. To date relatively few studies 92 have coupled metagenomics with a trait-based framework to clarify the drivers of community 93 assembly (Burke et al., 2011; Chen et al., 2008; Mackelprang et al., 2011; Raes et al., 2011). Even 94 fewer (indeed, none that we are aware of) have deployed such approaches in the context of whole- 95 ecosystem experimentation to test ecologically relevant hypotheses under field conditions. We focus 96 on a set of four information processing traits hypothesized to affect the ability of organisms to 4 97 obtain high maximum growth rates necessary for thriving in copiotrophic environments or reduce 98 the energetic and resource requirements necessary to persist under nutrient-poor conditions: 99 1. Multiplicity of genes essential to protein biosynthesis. Copiotrophs are predicted to have higher copy 100 numbers of rRNA operons and tRNA genes, since higher numbers of these genes increase 101 their maximum overall transcription rates, helping maintain higher abundances of ribosomes 102 (which are constructed from rRNA and proteins) and tRNAs. In turn, the larger pools of 103 ribosomes and tRNAs facilitate increased translation rates of protein synthesis necessary for 104 achieving high growth rates (Condon et al., 1995; Higgs and Ran, 2008; Rocha, 2004; Roller et 105 al., 2016). However, higher numbers of these genes incur costs, potentially putting organisms 106 with more copies of rRNA and tRNA genes at a disadvantage under low resource 107 conditions. In particular, more DNA has to be synthesized, maintained, and regulated, and 108 there may be an increased risk of transcribing overly large phosphorus-rich pools of rRNA 109 and tRNA, increasing phosphorus requirements (Elser et al., 2003, 1996; Godwin et al., 110 2017; Makino et al., 2003) and reducing growth efficiency (Roller et al., 2016). 111 2. Genome size. Organisms with smaller genomes are predicted to do better in stable and 112 oligotrophic environments, as they require fewer resources (such as phosphorus, P) to 113 maintain and replicate their genomes, have higher carbon use efficiency (Saifuddin et al., 114 2019), and have smaller cells with increased surface-area-to-volume ratios facilitating 115 resource uptake (Giovannini 2015). In contrast, organisms with larger genomes should do 116 better in complex or copiotrophic environments, where they can take advantage of their 117 typically higher intrinsic growth rates (DeLong et al., 2006) and more diverse and flexible 118 gene and metabolic networks (e.g., Konstantinidis
Details
-
File Typepdf
-
Upload Time-
-
Content LanguagesEnglish
-
Upload UserAnonymous/Not logged-in
-
File Pages45 Page
-
File Size-