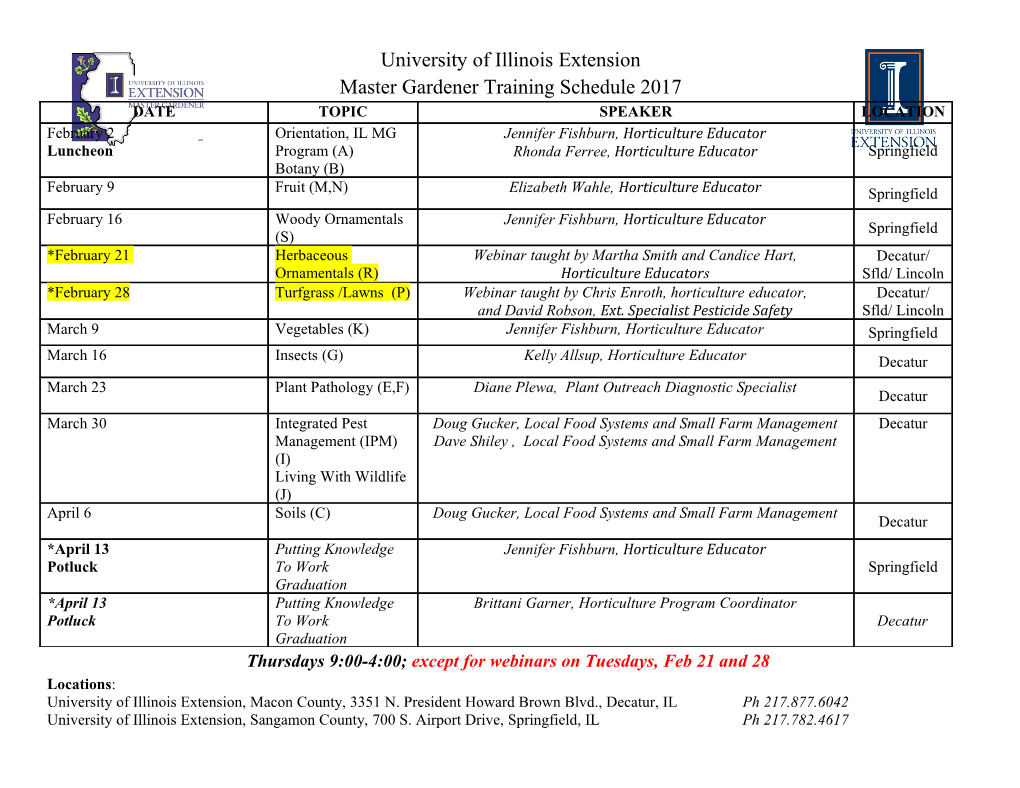
Hyperfine Interact (2010) 196:143–150 DOI 10.1007/s10751-009-0153-z Status of the LASER-IGISOL collaboration at the University of Jyväskylä F. C. Charlwood · K. Baczynska · J. Billowes · P. Campbell · B. Cheal · T. Eronen · D. H. Forest · T. Kessler · I. D. Moore · R. Powis · M. Reponen · M. Rüffer · G. Tungate · J. Äystö Published online: 20 January 2010 © Springer Science+Business Media B.V. 2010 Abstract Recent laser spectroscopy studies at the IGISOL facility, University of Jyväskylä have focussed on the shape transition at N = 60. A new technique of optical pumping in the ion beam cooler has given unique access to the radioactive isotopes of niobium and yttrium. Further spectroscopy from metastable states al- lowed nuclear moments and charge radii to be extracted for isotopes in the chains of Mo and Nb, thus exploring the extent of nuclear deformation across the entire Z = 36–42 region. Keywords Laser spectroscopy · Nuclear charge radii · Ion beam coolers 1 Introduction Laser spectroscopy provides measurements of the spins, nuclear moments and mean- square charge radii of ground and isomeric states of radioactive nuclei [1, 2]. Beams of all elements including refractory elements are produced for study at the IGISOL facility and can be prepared for efficient optical spectroscopy [3]. Following a fast extraction and mass analysis, ion beams are injected into an RF quadrupole trap [4] which acts to cool and bunch the ensemble. The beam emittance and energy spread are reduced, and the photon background in the subsequent spectroscopy is suppressed by use of a bunched beam [5]. F. C. Charlwood (B) · J. Billowes · P. Campbell · B. Cheal The University of Manchester, Schuster Building, Brunswick Street, Manchester, M13 9PL, UK e-mail: [email protected] K. Baczynska · D. H. Forest · R. Powis · M. Rüffer · G. Tungate School of Physics and Astronomy, The University of Birmingham, Birmingham, B15 2TT, UK T. Eronen · T. Kessler · I. D. Moore · M. Reponen · J. Äystö Department of Physics, University of Jyväskylä, Jyväskylä, Finland 144 F.C. Charlwood et al. Laser spectroscopy on cooled ionic beams primarily uses the excitation of ions from the ground state or naturally populated metastable states. Resonantly illuminat- ing the axis of the cooler-buncher using a broadband, high power titanium sapphire (Ti:Sa) laser can drive optical transitions from the ionic ground states and is used to enhance a selected metastable state [6]. Many ground state transitions are poorly suited to collinear spectroscopy; the transition may lie outside of the high resolution cw laser wavelength range or be simply inefficient despite a large population. Here, following population manipulation, efficient spectroscopic transitions can be chosen on the basis of strength, wavelength, spin or hyperfine structure. 2 Optical pumping in the N = 60 region Previous measurements [5, 7] by our collaboration concentrated on studies of yttrium and zirconium isotopes in the region of deformation around N ≈ 60.Herethe addition of neutrons above the N = 50 shell closure results in an increasing oblate shape and increasing β softness until N = 60. At this point, a distinct transition to a rigid prolate deformation occurs, with the effect most pronounced in the Y isotopes. Previous spectroscopy of Y [7] employed a ground state transition and the extraction of nuclear parameters were dependent on conditional spin assignments (unknown in 98mY, 100Yand102Y). A J = 0 ground state, as in the cases of singly charged Nb and Y ions, limits all optical transitions to J = 0 → J = 1 and restricts the extraction of nuclear moments and mean-square charge radii. Higher J transitions must be studied to determine the nuclear spin. Ground state transfer of the population in yttrium was attained using ≈30 mW of frequency-doubled Ti:Sa light at 363.4 nm. Spectroscopy from the 1,045 cm−1 state, populated by the pumping, allowed a spin confirmation to be made in 100YonaJ = 2 → J = 1 transition. In Nb, the ground state transition at 286.6 nm was utilized to indirectly populate the metastable level at 2,357 cm−1 for efficient spectroscopy on a 290.9 nm transition. Measuring hyperfine resonances in Nb would be impossibly inefficient without this new technique [6]. Non-optical data or theoretical estimates of atomic parameters are needed to calibrate the electron fields used in the extraction of nuclear data. In cases where an element has only one stable isotope, e.g. Y and Nb, only theoretical estimates exist for such calibrations. These calculations are relatively simple when using pure transitions but unfortunately such cases are rarely available for study. In the alkali like Y2+ ion, a pure “s → p” transition at 294.4 nm exists but the lowest 4s configuration does not form the ground state. Access to this transition was gained by optical pumping the 232.8 nm ground state line to populate the required 7,467 cm−1 metastable level. In all cases, the radially confined, cooled ions drift slowly along the cooler-buncher under the influence of an axial DC field. The slow motion increases the interaction time with the broadband lasers at the end of the cooler where pumping of electronic level populations take place (see Fig. 1). Experimental confirmation that this point alone is where the observed pumping occurs was achieved during the work on yttrium. In these studies, an entire ensemble of 89Y+ ions had their ground state depopulated with pulsed Ti:Sa light illuminating the cooler axis on a 363.3 nm ground state line. This confirms that the point of the laser-ion interaction must be inside the Status of the LASER-IGISOL collaboration at JYFL 145 Fig. 1 Optical pumping setup showing the titanium sapphire laser beams being directed along the axis of the cooler-buncher. After extraction from the RFQ, the ion beam is deflected by a quadrupole bend to the laser spectroscopy station cooler as only ≈30% of bunched ions would temporally overlap if the effect were occurring outside the gas cell. New studies using pumping in the quadrupole bend section, with synchronised trap and laser switching, are underway to investigate the relaxation of state population and spectroscopy from high lying metastable states. 3 Spin determinations in Nb Isotopes of niobium were produced at the IGISOL in the fusion evaporation reactions 93Nb(p,pxn)90,90m,91,91m,92Nb at 33 MeV and in the 30 MeV proton induced fission reactions on natU. As described above, optical pumping on the J = 0 → J = 1 line at 286.6 nm greatly improved the efficiency of spectroscopy on the 290.9 nm transition (J = 1 → J = 1)[6]. The measurement of nuclear spin in the 101,103Nb isotopes, which occur beyond the N = 60 shape change, were targeted as priority. The results of the χ 2 fitting for the possible spin assignments are shown graphically for 101Nb (Fig. 2) and in Table. 1.Aχ 2 minimisation technique was used to fit Lorentzian profiles to the 101,103Nb structures with nuclear spins of I = 3/2 → 9/2. An I = 1/2 assignment would produce a hyperfine splitting with four peaks, instead of the five observed, and could be ruled out. Spins greater than 9/2, the maximum spin observed before the shape change, produce fits of identical quality. Hyperfine 146 F.C. Charlwood et al. 20 15 Fit for I=3/2 2 χ =176.7 10 5 200 15 Fit for I=5/2 2 χ =71.1 10 5 200 Counts 15 Fit for I=7/2 2 χ =138.1 10 5 200 15 Fit for I=9/2 2 χ =148.4 10 5 0 -8000 -6000 -4000 -2000 0 2000 91g Frequency (MHz) relative to Nb Fig. 2 Resonance spectra and associated fits for a range of spin assignments in 101Nb. The disparity in the fitting for spins not equal to 5/2 is easily seen in the middle doublet structure (shown in the zoom inset) Table 1 A range of spin 92Nb 103Nb assignments in 92,103Nb with χ 2 χ 2 their respective χ 2 values I I derived from the fitting 6 139.1 3/2 268.3 process 7 114.5 5/2 117.6 8 134.9 7/2 207.1 9/2 187.5 A and B coefficients were held in the ratio of upper to lower states, using the stable 93Nb hyperfine parameters as a calibration. Three free parameters (A and B of the upper state and the structure centroid) must then fit the entire structure. The effect of restraining these parameters to fit the outer hyperfine peaks, in Fig. 2, results in the fitting of the middle doublet of the structure as shown. Peak intensities, FWHM and the backgrounds of each data set were determined from “free” fitting the structure. In both isotopes, the χ 2 confidently assigns a nuclear spin of I = 5/2. As shown in Table. 1,theχ 2 in 103Nb is lower for 9/2 than 7/2, contrary to expectation. This is because the calculated peaks for the middle doublet (with a similar structure to 101Nb) are outside of the scan range of the experimental data, with no contribution to χ 2. A similar process in 92Nb yielded I = 7, see Table 1. Magnetic and quadrupole moments were then extracted for each isotope. Status of the LASER-IGISOL collaboration at JYFL 147 400 91,90 200 0 -200 Modified Nb IS 91,92 -400 91,93 -600 -800 -0.1 0 0.1 0.2 0.3 0.4 2 Modified Zr δ<r > Fig. 3 A modified King Plot of Nb isotope shifts plotted against δr2 of the Zr chain [9] The isotope shifts (δvA,A ), measured along the Nb chain between isotopes A and A , are related to the mean square charge radii (δr2A,A )by, − A,A A A 2 A,A δv = M + F δr .
Details
-
File Typepdf
-
Upload Time-
-
Content LanguagesEnglish
-
Upload UserAnonymous/Not logged-in
-
File Pages8 Page
-
File Size-