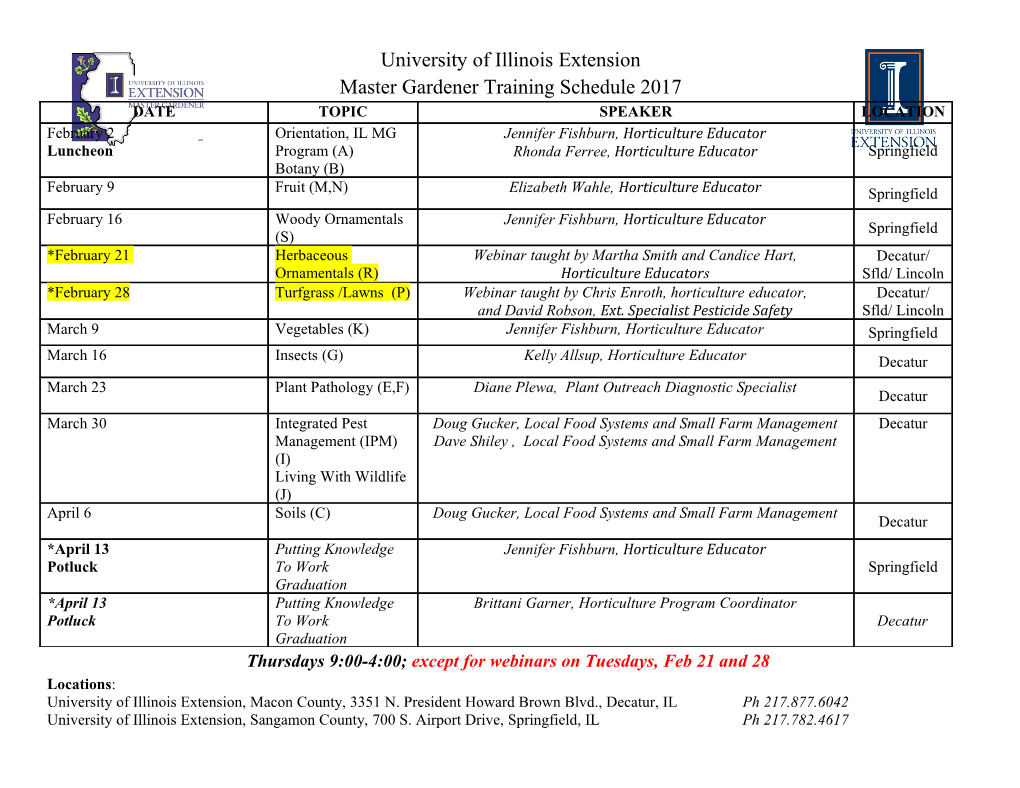
GENETICS AND PATTERNS OF INHERITANCE Simon Petersen-Jones DVetMed PhD DVOphthal MRCVS DECVO Department of Small Animal Clinical Science Michigan State University Veterinary Medical Center 736 Wilson Road, D-208 East Lansing. MI 48824 [email protected] INTRODUCTION The genome contains a complete set of instructions to make an organism and control its cellular structures and activities. This information, or genome, is contained within tightly coiled threads of deoxyribonucleic acid (DNA) found in the nucleus of every cell. The DNA is divided into chromosomes. The human genome consists of about 3 billion basepairs (Mb) (dog genome consists of 2.4Mb) and disease may result from an alteration of just one of those basepairs. It is estimated that the human genome contains in the region of 20, - 25,000 genes, whereas the dog is estimated to have only ~19,000 genes. Each gene carries the code for making a particular protein. Only a subset of the total number of genes will be functional in a particular cell type. Certain genes, known as house-keeping genes are expressed in all cell types and are needed for cell function. Other genes are tissue or cell-type specific. Although 20 –25,000 may seem a small number of genes to encode for and control the functions of a complex organism, further complexity if provided by a process of alternative spicing of genes. Alternative splicing between different tissues allows the same gene to produce differing proteins dependent on the tissue in which the gene is active. From http://www.exonhit.com/alternativesplicing/index.html - see this web page for more information on alternative splicing. Disease states are all influenced by genetic makeup. Some conditions result primarily from genetically determined factors (e.g. gene mutations). Although the animal’s genes (genotype) may dictate that it develops a disease, the actual characteristics of the disease (disease phenotype) i.e. how the disease is expressed in that individual (such as age of onset, rate of progression) may be influenced by environmental factors. Conversely diseases that at first glance appear totally environmental, such as infections with microorganisms are in fact influenced by genetic factors (e.g. factors that confer disease resistance). The situation may be further complicated when it is appreciated that expression of even simply inherited traits can be modified by “background genetics”. Basically this means that a variation (mutation) of one gene is the cause of the disease, but DNA variations elsewhere (at a modifying locus, or loci) influence the expression of the disease trait. 1 Perhaps the modifying locus encodes a protein that interacts with the disease protein or can render the animal relatively resistant to the effects of the mutation. DNA STRUCTURE DNA strands consist of repeating nucleotide units consisting of a phosphate group, a sugar (deoxyribose for DNA) and a base (adenine, cytosine, guanine or thymine). The DNA molecules consist of 2 strands that wrap around each other as a double helix. The two strands are joined by hydrogen bonds between the bases. Adenine (A) joins to thymine (T) and cytosine (C) to guanine (G) and thus the two strands are complementary to each other. 2 Note the different directionality of each strand – one runs from 5’ to 3’ and the complementary strand runs from 3’ to 5’. 3’ or 5’ refers to the number of the carbon atom of the deoxyribose sugar that forms a phosphodiester bond with the next sugar, as shown below. Folding of DNA to form a chromosome: The double helix of DNA is wrapped around 8 histone proteins in 2 loops. The length of the double loop is 146 nucleotides. This structure is known as a nucleosome. The DNA strand between adjacent 3 nucleosomes is about 60 basepairs long. The nucleosomes in turn wind into a cable 3 times thicker than the individual nucleosome. Each turn of the cable has about 6 nucleosomes. This cable is known as a chromatin fiber and this is resolvable by EM. During the metaphase stage of cell division the chromosomes become condensed. The chromatin fibers are looped around a central scaffold. Each loop is about 75,000 bp (75kb) long. These are then coiled to form the chromatids. NB: The significance of the nucleosome is that when a cell undergoes apoptosis (programmed cell death) the inter nucleosome DNA is cut. This results in cut sections of DNA each of which is a multiple of 180bp. This can be demonstrated on an agarose gel where a series of bands at 180bp, 360bp, 540bp…… can be seen, and indicates that apoptosis has occurred. Apoptosis plays a crucial role in the development of many structures including the retina. For example, developing retinal neurons that do not develop neuronal connections will be disposed of by apoptosis. Apoptosis also plays a role in death of cells during disease processes. Examples of this are death by apoptosis of photoreceptors of animals suffering from hereditary retinal dystrophies (e.g. PRA), and of ganglion cells in glaucoma. Several pathways can feed into the end result of apoptosis of the cell. There are currently investigations of experimental treatments to inhibit apoptosis as a way of saving photoreceptors in animals suffering from hereditary retinal dystrophies. CHROMOSOMES The DNA of the genome is divided into portions – the chromosomes. The nuclei of non-gametes have a diploid (2n) number of chromosomes. This means that there are 2 copies of each chromosome. The gametes (ova or sperm) are haploid (n) and have one copy of each chromosome. A normal diploid genome consists of two sex chromosomes and a number of autosomes (non-sex chromosomes). The number of autosomes varies between species. Chromosome number of different species: Species Diploid number Human 46 Dog 78 Cat 38 Horse 64 Donkey 62 Sheep 54 Cow 60 Pig 38 Rabbit 44 Mouse 40 The ends of the chromosomes are known as telomeres. These have repeated DNA elements and act to protect the chromosome from degradation during DNA replications. GENES Genes are lengths of DNA that code for individual proteins. The coding portion of genes only makes up a small percentage of the total DNA of the genome (c. 5%). The coding portions of the genes are split into segments called exons separated by noncoding intervening sequences called introns. The coding of genes is discussed under translation below. NON-CODING DNA The non-coding DNA serves many functions some of which are only relatively recently recognized. In addition to non-coding RNA such as transfer-RNA and ribosomal-RNA, microRNAs and long non-coding RNA has been identified. Micro-RNAs have a role in controlling translational activity of some genes. The functions of long non-coding RNAs (of which there are thousands) are not fully understood but some have been shown to have regulatory roles in gene transcription and mechanisms such as imprinting and X-chromosome inactivation. Having previously been described as “junk DNA” the functions of these non-coding regions and role in disease processes are becoming better understood. 4 TRANSCRIPTION & TRANSLATION The copying of DNA of a gene into RNA is called transcription. The messenger RNA transports the genetic message out of the nucleus to the cytoplasm where proteins are made by the translation of the genetic code. The mRNA codes for a specific order of specific amino acids. From the start site of translation the code is read in three basepairs units (a codon), one per amino acid. This coding can be likened to a series of three letter words. The following shows the effect if one letter (basepair) is missing, this is known as a deletion and results in a reading frame shift (the message is incorrect following the site of the deletion): Wild type: THE ONE RED EYE WAS OUT TOO FAR 1 basepair deletion: THE ONR EDE YEW ASO UTT OOF One amino acid may be coded for by up to 4 different triplets of basepairs (codons). The first two basepairs of the codon coding for any given amino acid are the same, the last basepair is the variable one (e.g. Alanine is coded for by: GCT, GCC, GCA or GCG). Three different triplets code for the end of the amino acid chain (stop codons TGA, TAG or TAA). RNA is in many ways similar to DNA except: It is single stranded The backbone sugar is ribose not deoxyribose RNA uses the pyrimidine base uracil where DNA uses thymine Transcription is a complex process under precise control with a number of proteins controlling the rate of transcription. These proteins interact with DNA sequences of the gene promoter. The entire system ensures that the appropriate protein is produced in the correct cell type and at the correct stage of life. The initiation of translation is when a TATA binding protein binds to a TATA sequence (TATA box) in the promoter. Other factors bind and interact with enhancer and silencer regions of the promoter. Finally RNA polymerase joins and transcription can start. The DNA is unwound and a complementary RNA copy is made of the coding strand of the DNA (the non-coding, complementary strand is not copied). The following shows a complementary strand of RNA: GGTTACCATTAACGAT - DNA sense strand CCAAUGGUAAUUGCUA - mRNA As mRNA is being formed the noncoding interruptions of the message (the introns) are spliced out. Introns start and end with a characteristic pair of bases (GT at the beginning and AG at the end). The intron/exon boundaries are known as the splice sites. A mutation at a splice site could mean that the intron is not removed from the mRNA or that an exon is cut out of the mRNA (either would completely alter the message).
Details
-
File Typepdf
-
Upload Time-
-
Content LanguagesEnglish
-
Upload UserAnonymous/Not logged-in
-
File Pages32 Page
-
File Size-