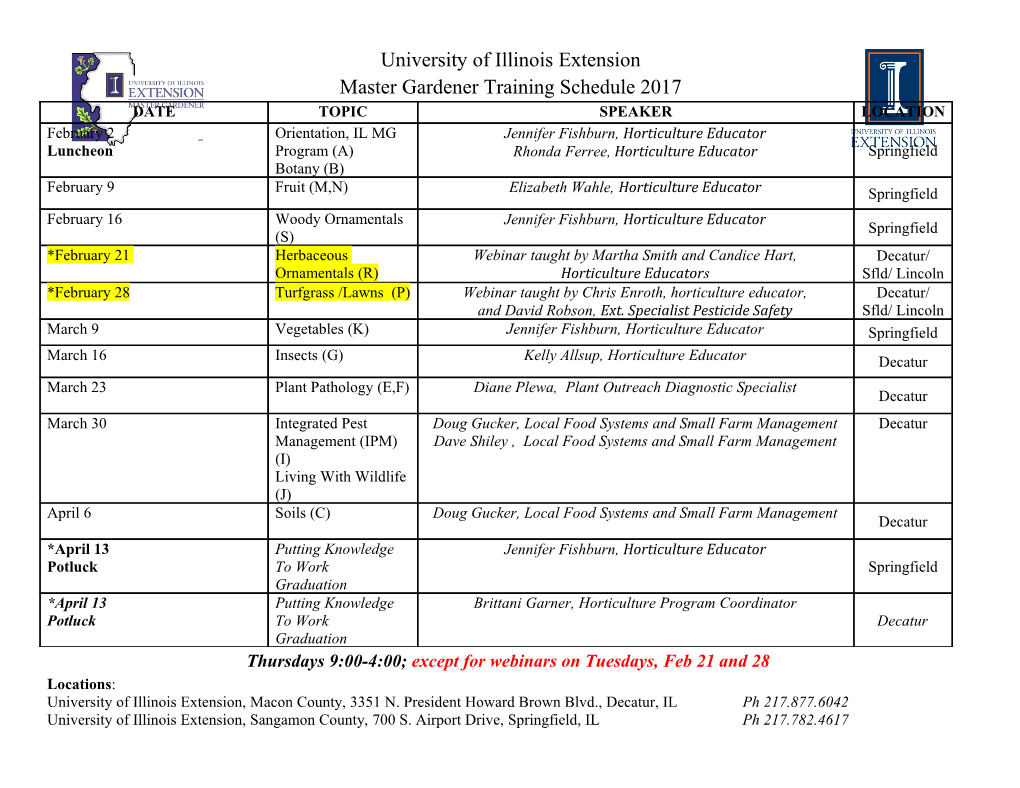
PHY418 Particle Astrophysics Susan Cartwright Contents 1 Introduction 7 1.1 Whatisparticleastrophysics?. 7 1.2 Early-universecosmology . 8 1.2.1 Inflation............................ 9 1.2.2 Baryogenesis ......................... 11 1.3 Thephysicsofdarkenergy . 17 1.4 High-energyprocessesinastrophysics. 20 1.4.1 The non-thermal universe . 21 1.4.2 Detectiontechniques . 22 1.5 Neutrinos ............................... 25 1.5.1 Neutrinosincosmology . 25 1.5.2 Solarneutrinos ........................ 26 1.5.3 Supernovaneutrinos . 26 1.5.4 Atmospheric neutrinos . 28 1.5.5 High-energyneutrinos . 28 1.6 Darkmatter.............................. 29 1.6.1 Astrophysical and cosmological evidence for dark matter . 29 1.6.2 Darkmattercandidates . 30 1.6.3 Detectionofdarkmatter . 34 1.7 Summary ............................... 40 1.8 QuestionsandProblems . 40 2 Astrophysical Accelerators: The Observational Evidence 43 2.1 Introduction.............................. 43 2.2 Cosmicrays.............................. 44 2.2.1 Abriefhistory ........................ 44 2.2.2 Detectionofcosmicrays . 45 2.2.3 Observedproperties . 56 2.3 Radioemission ............................ 69 2.3.1 Theradiosky......................... 69 2.3.2 Radio emission mechanisms . 71 2.3.3 Electromagnetic radiation from an accelerated charge . 73 2.3.4 Bremsstrahlung. 76 2.3.5 Synchrotron radiation . 80 2.3.6 Selfabsorption ........................ 86 2.3.7 Summary ........................... 88 2.4 Highenergyphotons ......................... 89 2.4.1 High energy photons and particle astrophysics . 89 2.4.2 Mechanisms of high-energy photon emission . 89 2.4.3 X-rays............................. 94 2.4.4 Soft γ-rays .......................... 97 2.4.5 Intermediate energy γ-rays .................103 3 4 2.4.6 High-energy γ-rays......................106 2.5 High-energyneutrinos . .118 2.5.1 Neutrino production and the Waxman–Bahcall bound . 119 2.5.2 Interaction of high-energy neutrinos with matter . 121 2.5.3 Detection of high-energy neutrinos . 123 2.5.4 Futureprospects . .126 2.5.5 Summary ...........................127 2.6 Anoverviewofsources. .127 2.6.1 Galacticsources . .128 2.6.2 Extragalacticsources. .131 2.6.3 Transientsources. .133 2.7 Summary ...............................137 2.8 QuestionsandProblems . .138 3 Astrophysical Accelerators: Acceleration Mechanisms 141 3.1 Introduction..............................141 3.2 The diffusion-loss equation . 142 3.3 Fermisecond-orderacceleration . 143 3.4 Astrophysicalshocks . .146 3.4.1 Shock jump conditions . 147 3.4.2 Theroleofcollisionlessshocks . 149 3.4.3 Effectofmagneticfields . .150 3.4.4 Observations of astrophysical shocks . 152 3.5 Diffusiveshockacceleration . .154 3.5.1 Test particle approach . 154 3.5.2 Beyond the test-particle approach . 158 3.5.3 Shockdriftacceleration . .161 3.6 Relativisticshocks . .162 3.7 Particle acceleration by magnetic reconnection . 165 3.8 Propagation of cosmic rays through the Galaxy . 167 3.9 Summary ...............................170 3.10 QuestionsandProblems . .172 4 Astrophysical Accelerators: Sources 175 4.1 Introduction..............................175 4.2 Particleaccelerationinthesolarsystem . 177 4.2.1 Solar flares and coronal mass ejections . 178 4.2.2 Planetarybowshocks . .179 4.2.3 The termination shock . 181 4.3 Galacticsources. .182 4.3.1 Supernovae and supernova remnants . 182 4.3.2 Evolution of a supernova remnant . 184 4.3.3 Observational evidence of particle acceleration . 186 4.3.4 Pulsarwindnebulae . .191 4.3.5 Evolution of pulsar wind nebulae . 193 4.3.6 ParticleaccelerationinPWNe . 194 4.4 Extragalacticsources. .198 4.4.1 Gamma-ray bursts . 199 4.4.2 Gamma-ray bursts as sources of UHE cosmic rays . 225 4.4.3 Radio-loud active galactic nuclei . 230 4.4.4 ParticleaccelerationinAGN . 243 4.5 Summary ...............................253 5 4.6 QuestionsandProblems . .258 6 Chapter 1 Introduction 1.1 What is particle astrophysics? Particle astrophysics, also known as astroparticle physics, is essentially the use of particle physics techniques, either experimental or theoretical, to address as- trophysical questions, or conversely the use of astrophysical data to constrain theories of particle physics. Examples of the former include gamma-ray as- tronomy and the development of the theory of inflation as an outgrowth from Grand Unified Theories; an example of the latter is the use of solar neutrinos to measure neutrino oscillation parameters. Particle astrophysics as a discipline in its own right is a relatively recent development, and the topics included under its umbrella vary from place to place. The journal Astroparticle Physics defines its subject matter as[1] High-energy cosmic-ray physics and astrophysics; • Particle cosmology; • Particle astrophysics; • Related astrophysics: supernova, AGN, cosmic abundances, dark matter • etc.; High-energy, VHE and UHE gamma-ray astronomy; • High- and low-energy neutrino astronomy; • Instrumentation and detector developments related to the above-mentioned • fields (a somewhat unsatisfactory definition since it includes “particle astrophysics” as a topic in its own right!). The Science and Technology Funding Council (STFC) defines particle astrophysics as “that branch of particle physics that studies elementary particles of astronomical origin, and their relation to astro- physics and cosmology” [2], but its description of the activities funded under this heading[3] includes gravitational waves, which do not seem to fit this def- inition. The 2008 and 2011 Roadmap documents of the Astroparticle Physics European Consortium (ApPEC) [4] define their subject as “the intersection of astrophysics, particle and nuclear physics and cosmology. It addresses questions like the nature of dark matter and dark energy, the physics of the Big Bang, the stability of protons, the properties of neutrinos and their role in cosmic evolu- tion, the interior of the Sun or supernovae as seen with neutrinos, the origin of cosmic rays, the nature of the Universe at extreme energies and violent cosmic 7 8 CHAPTER 1. INTRODUCTION processes as seen with gravitational waves.” The table of contents of the 2011 roadmap includes, as chapter or section headings, charged cosmic rays; • gamma-ray astrophysics; • high-energy neutrinos; • dark matter; • neutrino mass measurements (direct and via double beta decay); • low-energy neutrino astronomy; • proton decay; • dark energy; • gravitational waves. • Despite the minor variations, a fairly coherent picture of particle astro- physics emerges from these definitions. Essentially, the core disciplines of par- ticle astrophysics are 1. early-universe cosmology; 2. the physics of dark energy; 3. high-energy processes in astrophysics; 4. neutrinos; 5. dark matter. I have omitted gravitational waves from this list, despite their inclusion by both the STFC and ApPEC, because neither their production nor their detection involves particle physics (essentially, this is a classical phenomenon described by general relativity). However, the special case of primordial gravitational waves, detected via the imprint they leave on the polarisation of the cosmic microwave background, does belong in particle astrophysics because of its relevance to early-universe cosmology. In the rest of this chapter, we will briefly introduce each of the topics listed above. The rest of the course, however, will focus almost exclusively on the third and fourth items. Particle cosmology and the physics of dark energy will not be discussed in detail because they are very technical theoretical topics which would require a whole module to cover in adequate depth, while dark matter is covered in PHY326/426 Dark Matter and the Universe [5], and therefore will only be summarised here. 1.2 Early-universe cosmology The temperature of the universe now, as measured by the cosmic microwave background, is 2.72548 0.00057 K [6]. Temperature scales as (1 + z), where ± z is the redshift (see PHY306/406 Introduction to Cosmology [7]), so the early universe was much hotter than this, and hence had higher characteristic energies (E k T , where Boltzmann’s constant k = 8.65 10−5 eVK−1). Big Bang ≃ B B × nucleosynthesis (see PHY306/406 and PHY320 Nuclear Astrophysics [8]) takes 1.2. EARLY-UNIVERSE COSMOLOGY 9 place a few minutes after the Big Bang, at temperatures of order 109 K and energies of order 0.1 MeV. Energies above a few MeV, corresponding to times before 1 s or so after the Big Bang, are too high for nuclear physics and fall into the domain of particle physics, so early-universe cosmology is in many ways an application of theoretical particle physics, and is often referred to as particle cosmology. 1.2.1 Inflation One of the first applications of theoretical particle physics to early-universe cosmology was the development of the idea of inflation [9, 10], which used the physics of Grand Unified Theories at very high energies (E 1016 GeV, t ∼ ∼ 10−35 s after the Big Bang) to drive a brief period of extremely rapid expansion (usually assumed to be exponential, a exp(Ht), although a steep power law, ∝ a tn where n > 1, will also work). Inflation was originally postulated to ∝ account for two observations which are otherwise difficult to reconcile with the classical Big Bang model: the fact that the universe is observed to have a flat (Euclidean) geometry, and the extremely high level of isotropy displayed by the cosmic microwave background (see PHY306/406 for further details). Inflation also accounts for the small ( 10−5) anisotropies of the microwave ∼ background, which arise from quantum
Details
-
File Typepdf
-
Upload Time-
-
Content LanguagesEnglish
-
Upload UserAnonymous/Not logged-in
-
File Pages291 Page
-
File Size-