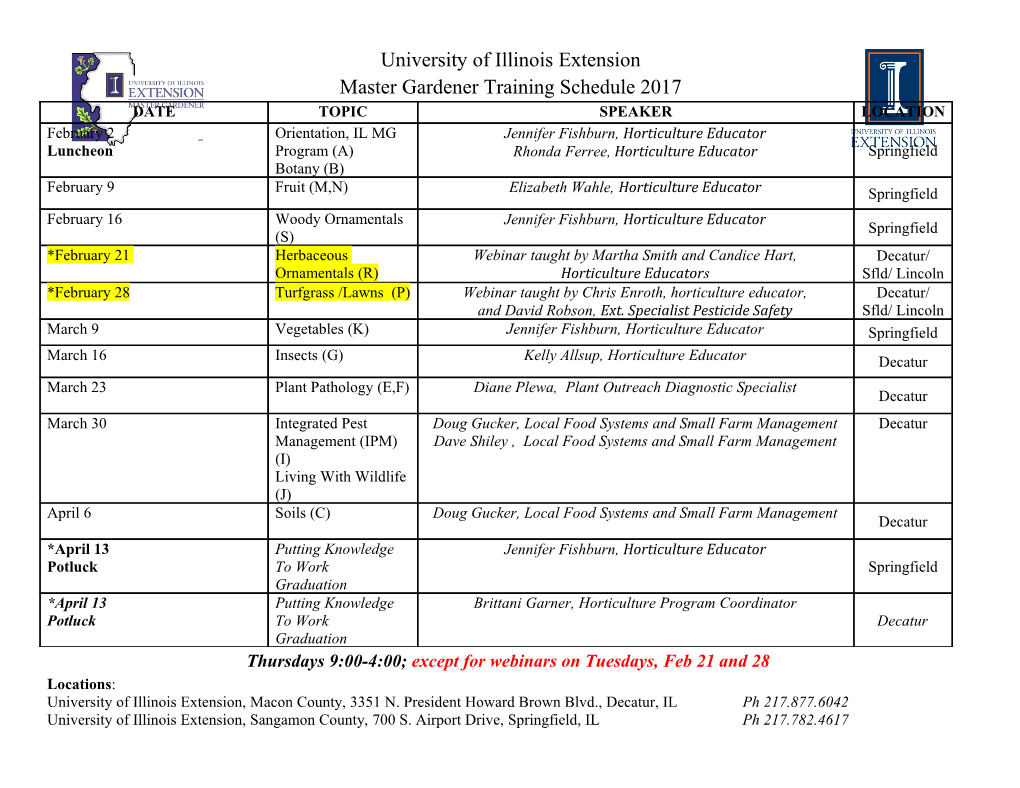
Letter pubs.acs.org/NanoLett Energy Transfer from Quantum Dots to Graphene and MoS2: The Role of Absorption and Screening in Two-Dimensional Materials † † ‡ ‡ ‡ Archana Raja,*, Andreś Montoya−Castillo, Johanna Zultak, Xiao-Xiao Zhang, Ziliang Ye, ‡ § § † † Cyrielle Roquelet, Daniel A. Chenet, Arend M. van der Zande, Pinshane Huang, Steffen Jockusch, § † † ∥ ⊥ James Hone, David R. Reichman, Louis E. Brus, and Tony F. Heinz*, , † ‡ § Department of Chemistry, Departments of Physics and Electrical Engineering, and Department of Mechanical Engineering, Columbia University, New York, New York 10027, United States ∥ Departments of Applied Physics and Photon Science, Stanford University, Stanford, California 94305, United States ⊥ SLAC National Accelerator Laboratory, Menlo Park, California 94025, United States *S Supporting Information ABSTRACT: We report efficient nonradiative energy transfer (NRET) from core−shell, semiconducting quantum dots to adjacent two-dimensional sheets of graphene and MoS2 of single- and few-layer thickness. We observe quenching of the photoluminescence (PL) from individual quantum dots and enhanced PL decay rates in time-resolved PL, corresponding to energy transfer rates of 1−10 ns−1. Our measurements reveal contrasting trends in the NRET rate from the quantum dot to the van der Waals material as a function of thickness. The rate increases significantly with increasing layer thickness of graphene, but decreases with increasing thickness of MoS2 layers. A classical electromagnetic theory accounts for both the trends and absolute rates observed for the NRET. The countervailing trends arise from the competition between screening and absorption of the electric field of the quantum dot dipole inside the acceptor layers. We extend our analysis to predict the type of NRET behavior for the near-field coupling of a chromophore to a range of semiconducting and metallic thin film materials. KEYWORDS: Energy transfer, quantum dots, graphene, MoS2, transition metal dichalcogenides, dielectric screening etallic, semiconducting, and insulating nanostructures the electric field of the donor dipole in the acceptor medium, are available as building blocks for novel optoelectronic and the dimensionality of the donor and acceptor.13 NRET M − and photonic devices.1 3 Near-field electromagnetic coupling plays an important role in the transfer of energy through the of these materials plays an important role in the design of such photosynthetic network of plants,14 solar cells,15 and quantum- devices. Colloidal semiconducting quantum dots (QD), which dot-based light-emitting diodes.2 Because the efficiency of exhibit high quantum efficiencies and seamless tunability of NRET is very sensitive to the separation between the donor bandgaps, are excellent absorbers and emitters of light. and acceptor, it is used to measure distances in biological However, QD films have relatively poor carrier transport systems16 and in nanoscale sensors with high specificity and low properties when compared to conventional semiconductors.4 In detection limits.17,18 Engineering the spectral overlap between contrast, graphite and the family of transition metal the donor and acceptor offers another way to control the dichalcogenide (TMDC) crystals, which are composed of NRET rate.19 The complex environments in photosynthetic two-dimensional (2D) layers coupled by weak van der Waals’ systems also require a greater understanding of the role of interactions, possess high mobilities.5,6 Consequently, bringing dielectric screening in tuning dipole−dipole coupling and the 20,21 these two classes of systems together for their respective rate of energy transfer. strengths and understanding the interaction of photoexcited With the emergence of two-dimensional materials like carriers in hybrid nanostructures is a burgeoning area of graphene and TMDC layers, there is a strong motivation, − research.7 11 both for fundamental reasons and as underpinnings for Nonradiative energy transfer (NRET) involves the transport potential applications, to extend our understanding of NRET of an excited electron−hole pair from an emitter or “donor” to to these novel systems. In particular, we wish to examine the an absorbing medium, or “acceptor”. The coupling is mediated prototypical case of energy transfer from a localized by near field interactions without the emission of photons and chromophore, such as zero-dimensional quantum dots, to can be understood as the quenching of the donor dipole in the these two-dimensional materials. Recently, there have been presence of a lossy medium.12 The efficiency of NRET depends on a number of factors, including the distance between the Received: December 8, 2015 donor and acceptor, the spectral overlap between the emission Revised: February 25, 2016 of the donor and absorption of the acceptor, the screening of Published: March 1, 2016 © 2016 American Chemical Society 2328 DOI: 10.1021/acs.nanolett.5b05012 Nano Lett. 2016, 16, 2328−2333 Nano Letters Letter 23 24 Figure 1. (a) Emission spectra of the QDs and the absorption spectra of monolayer graphene and MoS2. Inset: Schematic representation of core−shell QD and the 2D layered material hybrid structure. (b,c) Wide-field photoluminescence images of QDs spin-cast on monolayer graphene μ (outlined by dashed blue lines) and MoS2 (dashed red), respectively. The scale bars in the upper right corners are 5 m in length. The contrast has been enhanced in the images to allow the quenched QDs on the monolayers to be discerned. (d,e) Statistics of the PL from hundreds of individual QDs for the two monolayers, revealing PL quenching of over 90% in both cases. fi Figure 2. (a) TRPL traces on graphene layers are shown in the top panel and on MoS2 in the bottom panel. Exemplary ts are overlaid on the data in solid blue and red lines. The gray trace corresponds to the SiO2 substrate and the instrument response function is the dashed black line. (b) The QD lifetimes as a function of thickness of 2D layers are shown. Each data point corresponds to an average decay lifetime over multiple samples; the error bars represent the standard deviation of the measurements. The gray dashed line at 5 ns denotes the average lifetime of the dots on the SiO2 substrate. The solid red and blue lines are guides to the eye. ffi ’ ffi independent reports of e cient NRET to graphene and MoS2 input of the materials dielectric functions is su cient to explain based on different experimental techniques and theoretical both the contrasting trends with the thickness of the 2D models.7,10,22,46 Chen et al.7 reported an increasing rate of acceptor layer and the absolute rates within a factor of 2. The NRET with increasing thickness of the acceptor material, while countervailing trends for the different material systems reflect Prins et al.10 identified the opposite trend. Our goal is to differences in the relative importance of the additional layers on examine and understand NRET to these 2D layers within a the absorption and screening of the local electric field. unified experimental and theoretical framework. Experimental Methods. CdSe/CdZnS core−shell quan- In this Letter, we report a direct experimental comparison of tum dots, provided by QD Vision Inc., were spin-cast from a fl decay rates of quantum dot uorescence when the dilute solution onto varying thicknesses of graphene and MoS2 chromophores are placed on graphene and MoS2 layers of layers. The underlying substrate was a silicon wafer covered by ff fi fi di ering thickness. The measurements are performed using a 285 nm SiO2 lm. The sample con guration is shown time-resolved photoluminescence (TRPL) and photolumines- schematically in the inset of Figure 1a. The radius of the QDs cence (PL) quenching of individual quantum dots on was determined to be 5.4 nm through transmission electron monolayer graphene and MoS2. The increased PL decay rate microscopy; and the thicknesses of the 2D layers were observed for graphene and MoS2 is attributed to NRET. Upon established by a combination of Raman spectroscopy, atomic increasing the thickness of the acceptor material from force microscopy, and optical contrast measurements. monolayer to the bulk, the decay rate for the graphene layers For the time-resolved PL measurements, we excited the increases, while that on MoS2 decreases. For a given thickness, quantum dots with the frequency-doubled output of a the rate is always found to be higher in the case of graphene. modelocked Ti:sapphire laser providing 100 fs pulses of We show that a classical electromagnetic model with only the radiation at a wavelength of 405 nm at low pump fluences 2329 DOI: 10.1021/acs.nanolett.5b05012 Nano Lett. 2016, 16, 2328−2333 Nano Letters Letter Figure 3. (a) Normalized rate of NRET from QDs to graphene and MoS2 as a function of layer thickness. The rate of NRET on the respective bulk crystals are used for the normalization. Inset: Absolute rates of PL decay, presented as in the main figure. (b) Numerical simulations of the electric field of a QD dipole inside the acceptor layers. The black arrows represent the Poynting vectors, indicating the near-field transfer of energy from the QD to the underlying layer. The electric field has been reduced by a factor of 2 for the case of bilayer graphene. (<1 μJ/cm2). We monitored the time evolution of the resulting lifetime data were fitted to a biexponential form (solid lines PL through time correlated single photon counting using a fast overlaid on the monolayer signals in Figure 2a) convoluted avalanche photodiode. The instrument response function has a with the instrument response function (IRF, dotted black line full width at half-maximum of 50 ps, which is appreciably in Figure 2a). The slower component of the biexponential smaller than our shortest measured lifetime. A similar detection decay is taken to have a fixed lifetime of 5 ns. Across all the scheme was employed to characterize the TRPL of isolated measurements, the typical contribution of this component is on colloidal QDs using a suspension of QDs in toluene.
Details
-
File Typepdf
-
Upload Time-
-
Content LanguagesEnglish
-
Upload UserAnonymous/Not logged-in
-
File Pages6 Page
-
File Size-