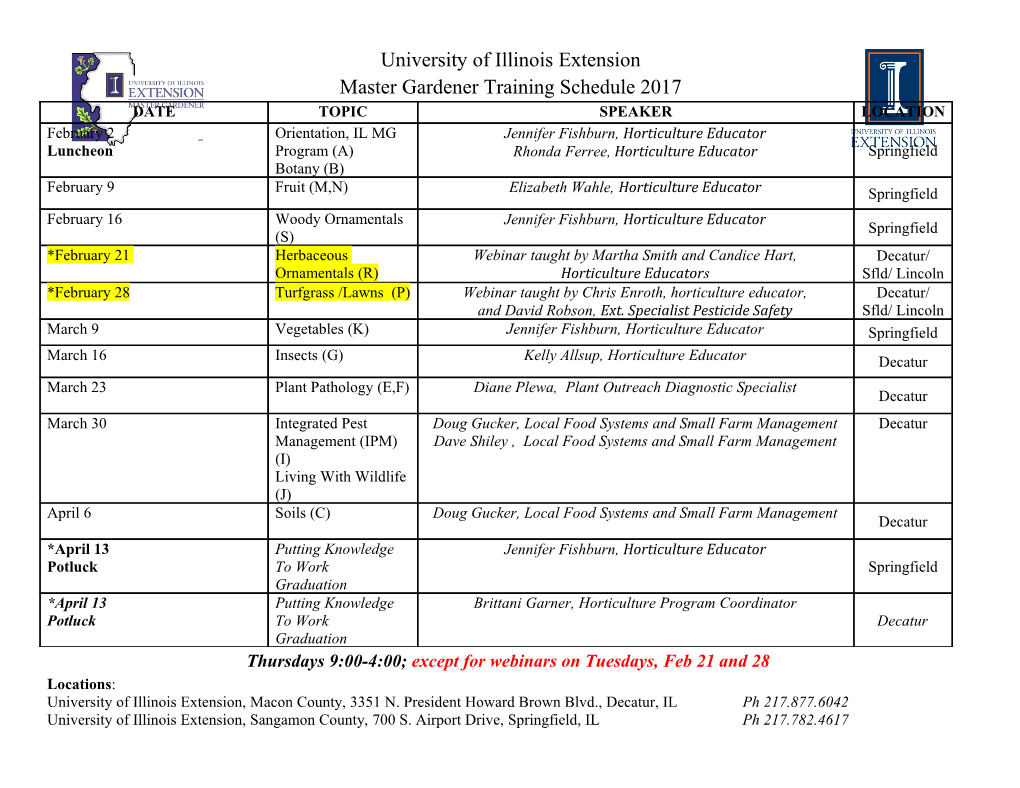
1 MECHANISM FOR DIFFERENTIAL DESICCATION TOLERANCE IN PORPHYRA SPECIES A Dissertation presented by Yen-Chun Liu to The Department of Biology In partial fulfillment of the requirements for the degree of Doctor of Philosophy in the field of Biology Northeastern University Boston, Massachusetts November, 2009 2 MECHANISM FOR DIFFERENTIAL DESICCATION TOLERANCE IN PORPHYRA SPECIES A Dissertation presented by Yen-Chun Liu ABSTRAC OF DISSERTATION Submitted in partial fulfillment of the requirements for the degree of Doctor of Philosophy in Biology in the Graduate School of Arts and Sciences of Northeastern University, November, 2009 3 Abstract Drought has been the cause of most agricultural losses, and therefore it is not surprising that desiccation has been one of the most studied stresses on plants. As a result, the mechanisms that desiccation tolerant terrestrial plants use to survive drought conditions are well understood today. This is not the case for those macrophytic marine algae (or seaweeds) that grow in the intertidal zone on rocky shores, which experience far more rapid and severe losses of water content than terrestrial plants. The goal of this study was to determine the mechanism of desiccation tolerance in seaweeds using the Red algae Porphyra umbilicalis and P. yezoensis as a model system. While these two algae have very different tolerances to desiccation, this study showed that both species lost about 95% of their water in the first two hours of dehydration and their final relative water content is virtually the same. Massive membrane leakage, reduced respiration and reduced oxygen evolution were observed in P. yezoensis after desiccation, but not in P. umbilicalis. TEM observation revealed extensive membrane disruption only in P. yezoensis after desiccation. Reactive oxygen species (ROS) defense, repression of membrane phase transition and formation of cellular glass are the three major desiccation tolerance mechanisms reported in land plants. ROS defense is not the key to the difference between P. umbilicalis and P. yezoensis for several reasons. First, desiccation in the dark did not alleviate the desiccation damage in P. yezoensis, as light has been shown to stimulate ROS damage. P. yezoensis also had higher superoxide dismutase activity. There was a small decrease in ascorbate content in P. umblicalis after desiccation, but such decrease 4 was not found in P. yezoensis. Furthermore, neither species showed an increase in membrane peroxidation after desiccation. Repression of membrane phase transition cannot explain the different response because the membranes of both species remained in liquid crystalline when desiccated. Our data suggest that the cytoplasm of P. umbilicalis forms a more stable glass when the organism is desiccated, and that the molecular mobility is lower in the drying P. umbilicalis. A dehydrin-like protein was detected in great abundance in P. umbilicalis and could play a key role in the better desiccation tolerance of this species. 5 Acknowledgements I would like to thank Dr. Donald Cheney for giving me the opportunity to conduct this study in his laboratory, for his patience, tolerance, and guidance throughout my studies. Otherwise, I could not finish this study. I would also like to thank my committee members: Dr. Cheney, Dr. Crowe, Dr. Scheirer, Dr. Bracken, and Dr. Vollmer for their advice throughout this research. I would like to thank the past members in the laboratory, including Andy, Angela, Jon, Chris, and Tim. Without their company, I can't go this far and stay sane. Most important of all, I would like to thank my parents, my sister, and my friends for their unconditional love and support throughout my life, especially in the past nine years. 6 Table of Contents Abstract 3 Acknowledgements 5 Table of Contents 6 List of Figures 8 List of Tables 10 Chapter 1: Introduction 11 A. The problems with drought and damage caused by desiccation 11 B. Proposed desiccation tolerance mechanisms in land plants 13 C. Knowledge about desiccation tolerance in macroalgae 18 D. Why Porphyra is a good model system of study of desiccation tolerance 31 E. Objective 33 Chapter 2: The role of ROS defense in desiccation tolerance of P. umbilicalis 35 Abstract 36 Introduction 37 Materials and Methods 40 Results 45 Discussion 48 References 55 Chapter 3: How intertidal seaweeds dry but don’t die: the mechanism of desiccation 7 tolerance in Porphyra 69 Abstract 70 Introduction 71 Results and Discussion 74 Materials and Methods 82 References 87 Chapter 4: Determination of saturation irradiance level of laboratory Porphyra cultures and characterizing field sample of P. umbilicalis and P. yezoensis 101 Objective 102 Materials and Methods 102 Results and Disccusion 105 Conclusion 108 Chapter 5: Discussion 123 References 130 8 List of Figures Chapter 2 Figure 1: Relative water contents of Porphyra species during dehydration 58 Figure 2: Effect of desiccation on photosynthesis 59 Figure 3: Effect of desiccation on respiration 60 Figure 4: Effect of dehydration membrane leakage 61 Figure 5: Effect of light on membrane leakage 62 Figure 6: SOD activities of Porphyra species during dehydration 63 Figure 7: Effect of desiccation on ascorbate contents 64 Figure 8: Effect of desiccation on peroxide concentration 65 Chapter 3 Figure 1: Absolute water contents of Porphyra species during dehydration 92 Figure 2: Effect of desiccation on phycobiliprotein fluorescence 93 Figure 3: Relationship between water content and membrane leakage 94 Figure 4: Effect of desiccation on thylakoid membranes 95 Figure 5: FTIR spectra of Porphyra species 96 Figure 6: Shift of νOH band with increasing temperature 97 Figure 7: Molecular mobility in the drying Porphyra species 98 Figure 8: Western blot of dehydrin-like protein in Porphyra species 99 Chapter 4 Figure 1: Oxygen evolution and P-I curve for laboratory-grown Porphyra yezoensis 110 9 Figure 2: Oxygen evolution and P-I curve for laboratory-grown Porphyra umbilicalis 111 Figure 3: Relative water contents of field collected Porphyra species during dehydration 112 Figure 4: Effect of dehydration membrane leakage of field collected Porphyra species 113 Figure 5: Superoxide dismutaste activities of field collected Porphyra species during dehydration 114 Figure 6: Western blot of dehydrin-like protein in laboratory-grown and field collected Porphyra species 115 10 List of Tables Chapter 1 Table 1: Review of studies on dehydration and desiccation of intertidal macroalgae 26 Table 2: Examples of studies on mechanisms of desiccation tolerance in macroalgae 30 Chapter 2 Table 1: ANOVA table for tests on relative water contents during desiccation, effects of desiccation on photosynthesis, respiration, amino acid leakage, ascorbate contents, and peroxide concentrations, and effects of light on amino acid leakage 66 Chapter 3 Table 1: ANOVA table for tests on water contents during desiccation, the effects of water contents on amino acid leakage, the effect of temperature on the shift of νOH stretch band and the effect of water content on molecular mobility 100 Chapter 4 Table 1: ANOVA table for tests on water contents during desiccation, the effects of water contents on amino acid leakage, and the effect of water contents on superoxide dismutase activity 116 11 Chapter 1 Introduction A. The problems with drought and damage caused by desiccation Water deficit and drought is the single most widespread factor that limits agriculture production (Chaves & Oliveira 2004). Boyer (1982) pointed out that drought affected more regions of the United States than any other environmental limitation. It also affects agriculture on every other continent (Kogan 1997). For example, in 2001 drought resulted in more than $5 billion dollars loss in agriculture in Canada (Phillips 2001), and it impacted 80% of the arable land of Henan, the most important province for winter wheat in China (R. Liu et al. 2006). Furthermore, drought is aggravated by human activities and global warming (Guobin & S. Chen 2006; Mittler 2006). Therefore, there is a great deal of interest in drought related issues in plant biology today (Chaerle et al. 2005; Parry et al. 2005; Flexas et al. 2006). Desiccation has several adverse effects on the cell. For example, dehydration of plant cells leads to the decrease of the availability of CO2 for photosynthesis and causes unbalanced photosynthesis (Bota et al. 2004; Flexas et al. 2006). In excess light, the unbalanced photosynthesis results in a high concentration of oxygen, a high level of photorespiration and excited chlorophyll molecules, which all in turn favor the accumulation of reactive oxygen species (ROS) (Smirnoff 1993). The high concentration of oxygen from photosynthetic oxygen evolution and low availability of CO2 enhance 12 photorespiration. Hydrogen peroxide is generated when glycolate, a byproduct of photorespiration, is metabolized (Bob B. Buchanan et al. 2000). When CO2 fixation is limited at low CO2 concentration, the levels of reduced electron carriers in the photosynthetic electron transfer chain increase and oxygen molecules are reduced by these electron carriers more easily. For example, reduced ferredoxin in photosystem I converts oxygen to superoxide in such conditions and NAD(P)H stimulates superoxide formation in microsomal, peroxisomal and glyoxysomal membranes (Smirnoff 1993). Also, singlet oxygen is generated when the excited chlorophyll molecule transfers its energy to the oxygen molecule and this ROS is believed to be more damaging to the membranes because it
Details
-
File Typepdf
-
Upload Time-
-
Content LanguagesEnglish
-
Upload UserAnonymous/Not logged-in
-
File Pages134 Page
-
File Size-