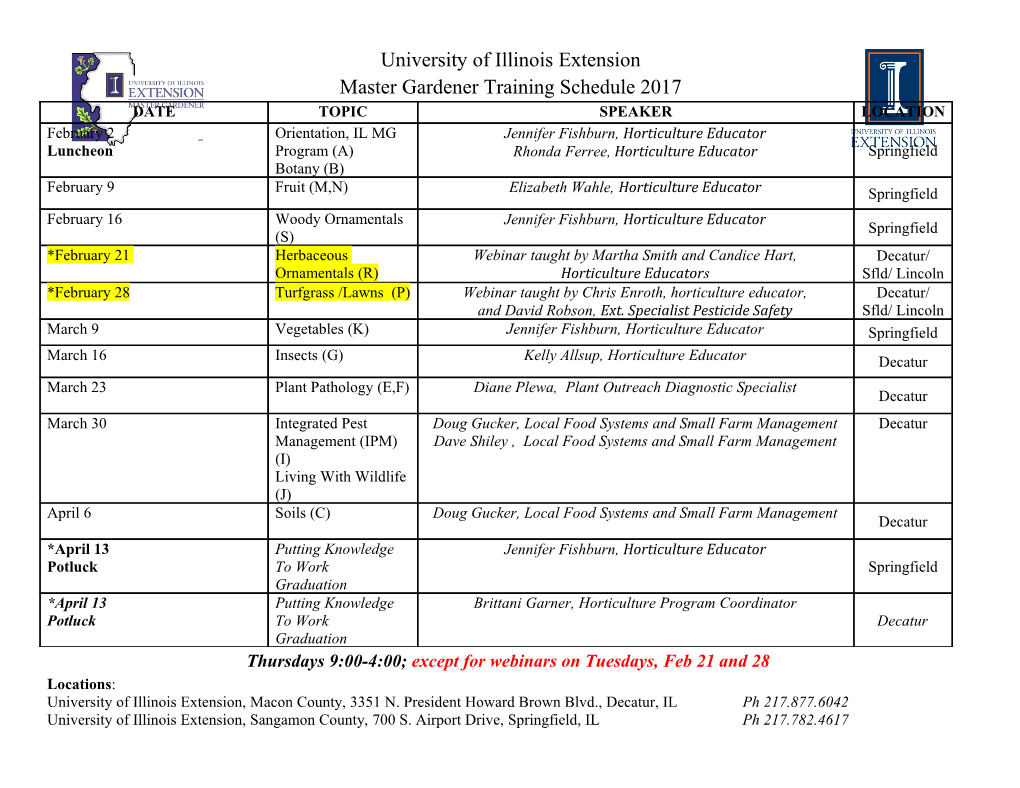
NORWEGIAN JOURNAL OF GEOLOGY Geometry of a major slump structure in the Storegga 1 Geometry of a major slump structure in the Storegga slide region offshore western Norway Roald B. Færseth & Bjørn Helge Sætersmoen Færseth, R.B. & Sætersmoen, B.H. 2008: Geometry of a major slump structure in the Storegga slide region offshore western Norway. Norwegian Journal of Geology vol 88, pp 1-11. Trondheim 2008. ISSN 029-196X. A gravitational collapse structure, 155 km long with a maximum width of 35 km, has been released as a single slope failure in the Storegga region off western Norway. The released volume of sediment, which we interpret as a slump, is a part of and of the same age as the Storegga Slide. This means that the slump was released approximately 8200 years ago. Unlike the main Storegga Slide, in which slide sediments have been totally disintegrated by debris flows, the slump was subject to relatively modest down-slope displacement and is an intact body located along the southern margin of the Storegga Slide scar. Rotational movement of the slump sediments released horizontal stress at the head of the slump and at the same time, horizon- tal stress intensified down-slope where contractional structures such as folds, reverse faults and thrusts developed. Roald B. Færseth, Hydro ASA, Research Centre, Box 7190, N-5020 Bergen, Norway. Present address: Ryenbergveien 75A, N-0677 Oslo, Norway (e-mail: [email protected]); Bjørn Helge Sætersmoen, Petroleum Geo-Services, P.O. Box 290, N-1326 Lysaker, Norway (e-mail: Bjorn.Helge. [email protected]) Introduction an approximately 300 km long backwall along the pres- ent-day shelf edge. A volume of some 3000 km3 of sedi- Gravitational collapse structures, often termed landslides, ment was removed leaving the slide scar (Haflidason et possess two essential features: a rupture surface (failure al. 2004; Bryn et al. 2005a). The run-out distance was surface, glide surface, detachment, décollement) and a 450 km for the debris flows and 800 km for the associ- displaced mass of sediment (Hampton et al. 1996). The ated turbidity currents (Haflidason et al. 2005). The slide displaced mass is the material that travelled down-slope. affected an area of 90.000 km2 and was released in an It commonly rests partly on the rupture surface, but it area where the average slope inclination was of the order might have moved completely beyond it. Moreover, the of 0.6-0.7°. Unstable sediments in the area were removed displaced mass may remain intact, be slightly to highly during one main slide event at approximately 8200 years deformed, or break up into distinct slide blocks. In some BP (Haflidason et al. 2004; Bryn et al. 2005a). cases, all or part of the mass completely disintegrates, producing a flow. Gravitational collapse structures are The present study is concerned with the morphology common in many settings around the world, both sub- and internal structure of a specific gravitational col- aerially and subaqueously. Such structures are typically lapse structure in the Storegga region, which has not associated with areas of instability where some sort of been described previously. Unlike the Storegga Slide triggering mechanism resulted in tectonic failure (e.g. proper where slide sediments have, to a large extent dis- Hampton et al. 1996). integrated, the allochthonous mass of sediment we now describe was subject to relatively modest down-slope The Storegga region off western Norway has been the displacement and is an intact body located along the site of several giant submarine slides (Evans et al. 1996; southern margin of the Storegga Slide scar (Fig. 2). High- Bryn et al. 2003; Haflidason et al. 2004; Sejrup et al. 2004; resolution 2D and 3D seismic data and Multibeam Echo Nygård et al. 2005; Solheim et al. 2005). The Storegga Sounder data from the seabed excellently displays the Slide (Fig. 1) of Holocene age is one of the largest known slump-like shape of the body as well as its internal defor- submarine slides and has been known since the 1970s mation structures and their spatial distribution. (Bugge 1983; Jansen et al. 1987). Over the last decade, a large number of publications have described the Storegga Slide. One main reason for this interest in the area is Gravitational collapse structures related to the Ormen Lange gas field, discovered in 1997, which is located within the scar of the Storegga Slide Gravitational collapse structures may range in length (Fig. 2). This situation raises concerns about the hazard from centimetres to hundreds of kilometres and affect to which the production installations would be exposed. both loose sediments and consolidated rocks under The Storegga Slide scar defines a depression bounded by subaerial as well as subaqueous conditions (Martinsen 2 R. B. Færseth & B. H. Sætersmoen NORWEGIAN JOURNAL OF GEOLOGY Fig. 1. Bathymetrical map of the southern Norwegian Sea and the Norwegian margin area with outline of the Storegga Slide. T T T Fig. 2. Bathymetric image of the Storegga Slide scar with outline of the slump in its present location along the southern margin of the slide scar (stippled blue line). See Fig. 1 for location of this figure. Late Pliocene-Pleistocene sediments of the North Sea Fan represent the southern boundary of the slump on the sea floor, whereas inliers of the older Tampen Slide (T) are located along the northern boundary. The Ormen Lange gas field (continuous blue line) is located within the Storegga Slide scar. NORWEGIAN JOURNAL OF GEOLOGY Geometry of a major slump structure in the Storegga 3 Fig. 3. Schematic illustration showing characteristic geometries associated with gravitational collapse structures (after Echel 1958). This idealized model shows the displaced unit to have a well-defined upper extensional zone (head) and a down-slope contractional zone (toe). This is because movement of the mass of sediment releases horizontal stress at the head and at the same time, horizontal stress increases towards the toe. 1994; Hesthammer & Fossen 1999; Canals et al. 2004 and the rupture surface is layer-parallel (Ramsay & Huber references therein). There is, however, a significant dif- 1987). ference in size between subaerial and subaqueous land- slides (e.g. Hampton et al. 1996). The largest subaerial Terminology slides displace a few tens of cubic kilometres of material. The terms “slide” and “slump” are referred to in the lit- By comparison the largest submarine slide known, the erature as features produced by various mass gravita- post-Pliocene Agulhas slide off South Africa has a vol- tional processes, but the use of the terms has often been ume of 20.000 km3 and is interpreted to have occurred imprecise. Most classification schemes make a distinc- as a single slope failure event (Dingle 1977). Submarine tion between slide and slump mechanisms although landslides can originate in nearly flat areas. Analyses have different definitions are proposed (e.g. Hampton 1972; shown that the force of gravity alone is not sufficient to Coates 1977; Woodcock 1979; Allen 1985; Stow 1986; be the sole cause of failure (e.g. Ross 1971; Hampton et Nemec 1990; Martinsen 1994; Hesthammer & Fossen al. 1978). Gravity failure is generally due to a triggering 1999; Lucente and Pini 2003, Canals et al. 2004). Slide mechanism provided by seismic shocks, rapid sedimen- masses are in general said to move on planar rupture tation, over-steepening of slopes, changes in pore pres- surfaces (translational movements) and show no or little sure, or extensional deformation (Morton 1993). internal deformation, whereas slump masses move on an upwards-concave rupture surface (rotational move- Despite the variation in scale as well as variation in lith- ments) and show a wide range of internal deformation ification of sediments affected, there is a striking similar- mechanisms. Following this definition the term slump ity in the overall geometry of many gravity-related fail- should be used for the displaced mass of sediment ures (Fig. 3). The area affected is commonly described as described in this paper. spoon-shaped or amphitheatre-like (e.g. Eckel 1958; Var- nes 1958; Lewis 1971; Brundsen 1973; Brundsen & Jones Models of gravitational collapse structures 1976; Martinsen 1994; Hesthammer & Fossen 1999, Idealized models of gravitational collapse structures (e.g. Canals et al. 2004). In cross section, the rupture surface Eckel 1958; Lewis 1971; Farrell 1984) show the displaced typically displays a concave upwards geometry. The sur- unit to have a well-defined upper extensional zone (head) face may form a flat that is bedding-parallel in its cen- and a down-slope contractional zone (toe) (Fig. 3). This tral part but cuts across bedding planes at the head and is because movement of the mass of sediment releases in some cases also at the toe to define ramps (e.g. Varnes horizontal stress at the head and at the same time, hori- 1958; Levis 1971; Schwarz 1982). The bedding-parallel zontal stress increases towards the toe. Rupture surfaces part of a rupture surface is typically confined to strati- below slumps may display substantial curvature or sharp graphic horizons that appear to be inherently weak either bends. As the slump moves down and away from the because of low sediment strength, high fluid pressure, or scarp past irregularities along the substrate, there must both. The term décollement is generally used only where be deformation in the sediments above the detachment 4 R. B. Færseth & B. H. Sætersmoen NORWEGIAN JOURNAL OF GEOLOGY (Ramsay & Huber 1987). For this reason, folds may sediments of the upper part of the Naust Formation. develop within the advancing slump, formed by bend- Sediments in the Storegga region are severely dissected ing of the sediments as they slip over non-planar rupture by slides, and repeated sliding during the last 1.7 mil- surfaces.
Details
-
File Typepdf
-
Upload Time-
-
Content LanguagesEnglish
-
Upload UserAnonymous/Not logged-in
-
File Pages11 Page
-
File Size-