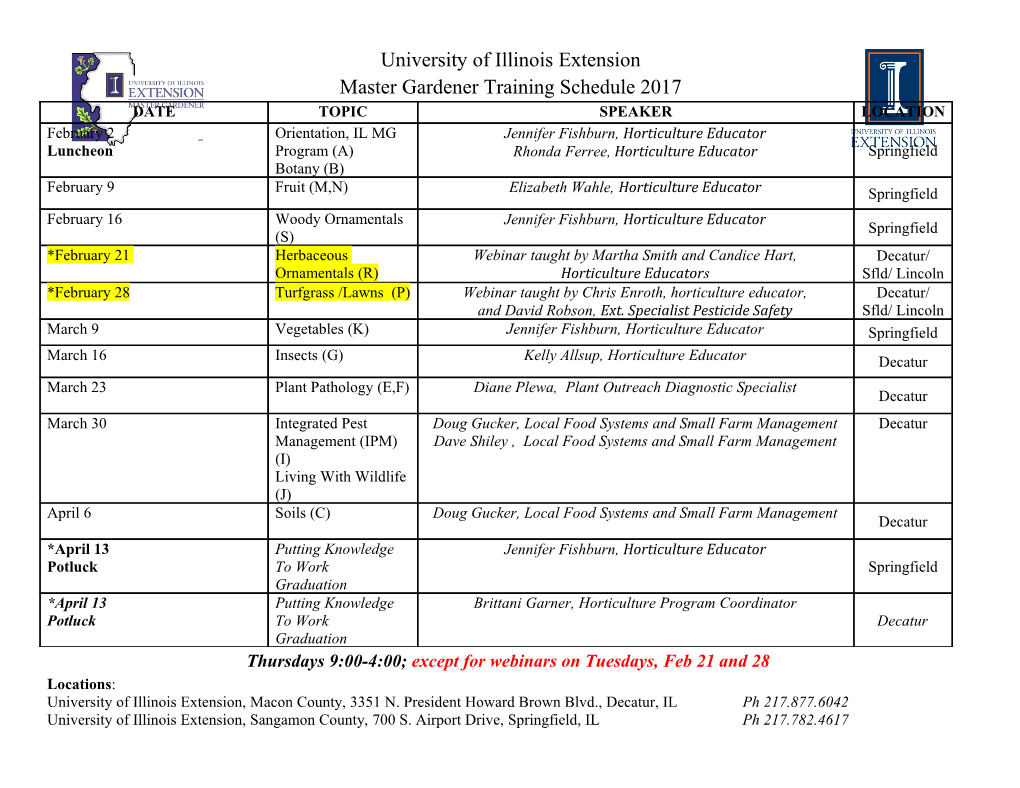
sensors Article Design and Experiments of a Piezoelectric Motor Using Three Rotating Mode Actuators Roland Ryndzionek * , Łukasz Sienkiewicz , Michał Michna and Filip Kutt Gda´nskUniversity of Technology, Faculty of Electrical and Control Engineering, 80-233 Gda´nsk,Poland; [email protected] (Ł.S.); [email protected] (M.M.); fi[email protected] (F.K.) * Correspondence: [email protected] Received: 17 October 2019; Accepted: 22 November 2019; Published: 26 November 2019 Abstract: This paper represents a numerical and experimental investigation of the multicell piezoelectric motor. The proposed design consists of three individual cells that are integrated into the stator, double rotor, and a preload system combined into a symmetrical structure of the motor. Each of the cells is characterized by a traveling wave and rotating mode motor. A finite element numerical analysis is carried out to obtain optimal geometrical dimensions of the individual cell in terms of generated vibrations and resonant frequencies of the structure. The results of the numerical analysis are compared with analytical calculations based on the equivalent circuit theory. Experimental tests are also presented, including laser interferometry measurements of vibrations generated at the surface of the stator, impedance analysis, as well as measurements of mechanical characteristics of the complete motor. The final stage of the study concludes that the presented motor can provide relatively high torque compared with other traveling wave rotary motors. Keywords: piezoelectric actuator; piezoelectric ultrasonic motor; finite element analysis; piezoelectricity 1. Introduction The subject of piezoelectric motors, sensors, and actuators has been, and still is widely discussed in the literature. Nevertheless, there is still great potential for the development of new structures and the improvement of existing ones. Some of the solutions have been commercialized and are used in the industry. The first invented and registered piezoelectric motor appeared in the USA in the 1940s [1]. In the following years, the great development of this technology could be observed. The industry acknowledged the enormous potential of the technology and invested huge amounts of resources on practical developments during the 1980s. Many new devices were introduced, including precision positioners with high strain materials, mass-produced multilayer devices for portable electronic appliances, ultrasonic motors for micro-robotics, and smart structures [2–4]. Economic stagnation and crisis in the 1990s slowed down the development of this technology. The exploration of new active materials slowed down as well. However, a multitude of new typologies and solutions have already been proposed in the twenty-first century [5–7]. In [3], Kenji Uchino wrote that we are currently experiencing a “renaissance” of piezoelectric actuators. The first research work on piezoelectricity was done by Carl Linnaeus and Franz Aepinus in the mid-18th century [8]. Their experiments included testing various materials, and examination of a voltage generated as an effect of temperature variations. This phenomenon was named pyroelectricity. Continuing that study, both René Just Haüy and Antoine César Becquerel posited a connection between mechanical stress and electric charge in some materials [9,10]. The term “piezoelectricity”, introduced by Wilhelm Gottlieb Hankel, describes the phenomenon of the induction of an electric charge or voltage in response to applied mechanical force or pressure to a piezoelectric material. Conversely, if Sensors 2019, 19, 5184; doi:10.3390/s19235184 www.mdpi.com/journal/sensors Sensors 2019, 19, 5184 2 of 19 some charge or voltage is imposed on a piezoelectric material, the material reacts by generating some mechanical force and strain [11]. This phenomenon is called the inverse piezoelectric effect. The direct piezoelectric effect was confirmed experimentally by Pierre and Jacques Curie in 1881. A year later, the Curie brothers demonstrated the existence of an inverse effect based on mathematical considerations and the thermodynamic research of Gabriel Lippmann [12–15]. Materials that exhibit a significant and useful piezoelectric effect fall into three main groups: natural and synthetic crystals, polarized piezoelectric ceramics, and certain polymer films. Ferroelectric ceramics are the most common piezoelectric material in today’s engineering applications. Among them, polycrystalline ceramics like barium titanate (BaTiO3) and lead zirconate titanate (PZT) are the most popular materials, in particular due to its low manufacturing costs and almost arbitrary shaping possibilities compared to single crystalline piezoelectrics. Piezoelectric ceramics are usually divided into two groups. The antonyms “hard” and “soft” doped piezoelectric materials refer to the ferroelectric properties, that is, the mobility of the dipoles or domains, and hence also to the polarization/depolarization behaviour. “Hard” piezoelectric materials are those materials whose properties are stable with temperature, electric field, and stress. They are used in applications requiring high power actuation or projection. The applications often have a narrow bandwidth, but are usually operated either at resonance or well under resonance. “Soft” piezoelectric materials are those materials whose properties have been enhanced for sensing, actuation, or both. They have high coupling and high permittivity. Property enhancement was made at the expense of the temperature, electric field, and stress stability [16]. Among the existing piezoelectric materials, hard PZT ceramics have the highest ability for application in the field of actuators, motors, or motion stages [17,18]. The comparison of chosen electromechanical transducer technologies and materials is introduced in Figure1. In the figure, the Y-axis describes specific driving efforts of transducers. This is the ability to produce the effort in terms of volume. The X-axis describes the relative speed of deformation. It represents the speed at which the transducer’s active part can be deformed and go back to its bulk. The product of these two quantities gives the theoretical power density. There is an observable distance in terms of specific driving efforts between the traveling wave motor and PZT ceramics. It clearly shows the remaining potential to be extracted from this electro-active material by novel actuator and motor designs. ] 103 MPa [ 102 101 100 10-1 -2 Specific driving efforts efforts driving Specific 10 10-2 10-1 100 101 102 103 Relative speed of deformation [rad/s] Hydraulic actuator Electromagnetic motor 1500 MW Biological muscle Shape-memory alloys Piezoelectric ceramics (PZT) Travelling wave motor Figure 1. A comparison of transducer technologies [19,20]. Maximum actuation force and velocity. Sensors 2019, 19, 5184 3 of 19 1.1. Classification of the Proposed Motor The two most common classification methods of piezoelectric motors are based on either the vibration type generated by the inverse piezoelectric phenomenon, or the output motion produced by a motor (Figure2). The widest group of piezoelectric motors in terms of vibration generation is resonant or ultrasonic motors [21,22]. An ultrasonic piezoelectric motor is one in which electrical energy is converted by the inverse piezo-effect to obtain displacement of the actuator at one of its resonant frequencies in the ultrasonic range. The displacements of the actuator results from excitation of different acoustic waves in the actuator shape. The waves generated by the actuator can be standing or traveling waves, depending on the number of contact points between the stationary and the moving part. On one hand, the use of standing waves (one point of contact) leads to a simpler mechanical structure of the motor and more basic driving circuitry, but on the other hand, results in limited output power [23]. Traveling wave motors generally have a larger power output, though at the expense of a more complex mechanical design. The motors operating outside resonance conditions fall into the group of quasi-static designs. Inertial quasi-static designs use the high and low speed profile of displacement, creating inertial motions of the motor. Structures using the inertial actuation mode can be designed as simple mechanical assemblies and driven with a single electrical source. This contributes to the notion that piezoelectric motors often have simpler and less complicated control systems than comparable electro-magnetic motors which do not require complex control strategies or a controller-observer approach [24–27]. Quasi-static walking motors use alternating motions of clamp and feed to advance the moving part step-by-step. The operation principle of this group was inspired by motions found in nature. The actuators using the walking mode have long strokes and high resolutions, but the whole motor structure and excitation signals are complex, due to the multiple groups of piezoelectric actuators [28]. Classification of piezoelectric motors based on the characteristics of the output motion describes the number of directions which motion piezoelectric structures can produce. The most common rotary and linear motors are single degree-of-freedom (DOF) designs. More complex structures are often preferable in micromechatronics systems, where multi-DOF motors are the optimal solution for reducing weight and volume [29]. Taking the above classifications into account (Figure2), the presented design is a single-DOF,
Details
-
File Typepdf
-
Upload Time-
-
Content LanguagesEnglish
-
Upload UserAnonymous/Not logged-in
-
File Pages19 Page
-
File Size-