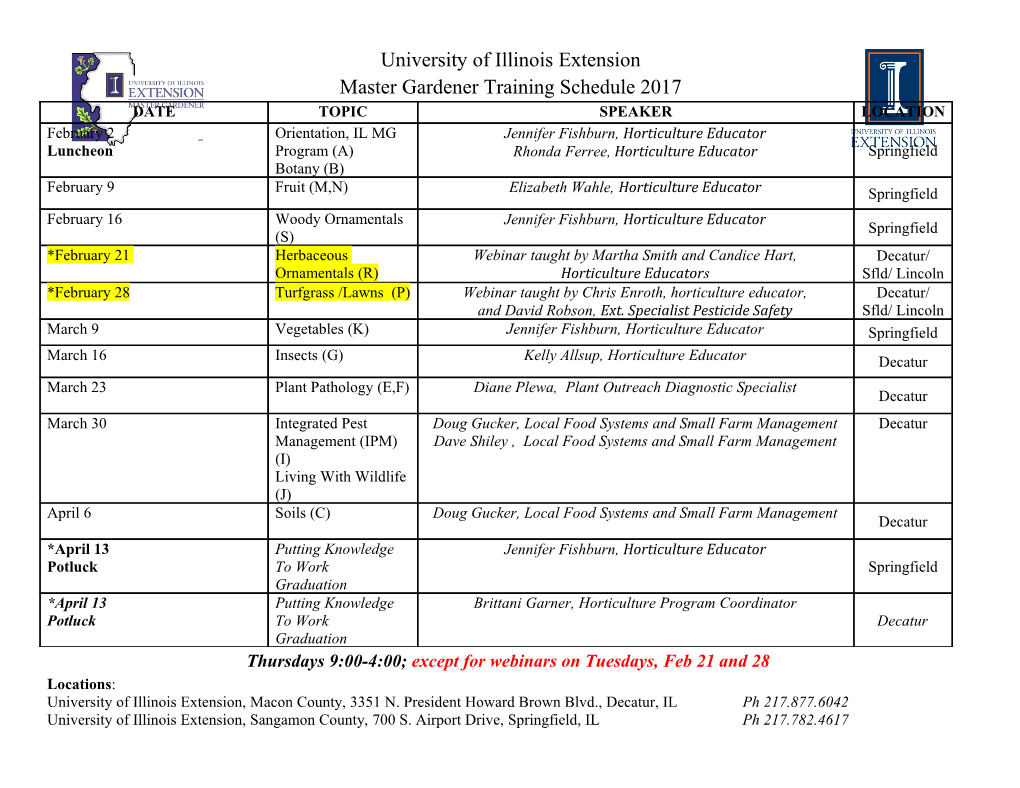
Tracking Light Oscillations: Attosecond Spectroscopy Comes of Age Ferenc Krausz Can time-resolved spectroscopy be extended into the attosecond domain to capture the motion of electrons in atoms? This article reviews recent research providing the basic tools for the emerging field of attosecond atomic spectroscopy and addresses what remains to be done to make the technique applicable to a broad range of processes and atomic systems. n 1887, Hertz observed that ultraviolet their concern might have been the ultra- have appeared impossible until recently, light that originates from an electrical high speed with which the strength of the the basic concepts underlying its recent discharge could be used to affect (or light field varies: half of a femtosecond implementation emerged before the end I 1 -15 influence) another discharge. This famous (1 fs = 10 s) or less elapses while the elec- of the nineteenth century. As early as 1866, experiment provided the first conclusive tric or magnetic field of visible light Töpler was able to capture the periodic evidence that light is an electromagnetic changes in strength from zero to its maxi- variation of atmospheric pressure in wave. But can the oscillations of a visible mum value or vice versa. Hence, to capture acoustic waves.2 To this end, he used a light wave be tracked and rendered directly the temporal evolution of light fields re- spark to induce a sound wave. This spark perceivable? If Hertz and his contempo- quires an attosecond sampling technique was also used to trigger the ignition of a raries had thought to ask this question, (1 as = 10-18 s). second spark through a delay line. The they might have been skeptical about a Although the development of an at- time-delayed spark emitted a flash of light positive answer. The most likely reason for tosecond sampling technique might well that recorded the instantaneous refractive- 62 Optics & Photonics News I May 2002 1047-6938/02/05/0062/7-$0015.00 © Optical Society of America ATTOSECOND SPECTROSCOPY Figure 1. All-optical sampling system. The ultra- short light pulse delivered by a collimated laser beam is split in two by a partially transmitting mir- ror. One of the two replicas serves as a pump Electrons, pulse to induce a transient change in the micro- Probe Pump Photons scopic structure of the investigated sample; the other delayed pulse probes the process triggered Beam Detector splitter by the pump pulse. The delay is most simply ac- complished by translating a mirror: an increase in Interaction the optical path length by 0.3 mm adds a 1000-fs medium delay.Changes in the microscopic structure are of- Delay ten probed more directly at frequencies (or wave- line lengths) that differ from those of the pump pulse. To this end, the carrier fre- quency (i.e., photon energy) of the pump and/or probe light can be shifted by frequency conversion techniques, ex- ploiting the nonlinear polariza- tion response of matter to in- tense radiation.The ultrashort duration of light pulses from mode-locked lasers benefits this generic scheme in several ways. First, it improves the temporal resolution of the sampling system. Second, the confinement of finite light en- ergy in a short time interval implies high peak intensities. This is crucial for efficient nonlinear frequency conver- sion of the pump or probe light and significantly enhances the versatility of the optical sampling system. index change of air induced by an acoustic ration of the pump and probe pulses (con- Figure 2. Motorcyclist in motion. The image is wave, from which the instantaneous den- sisting of the first and second spark, re- blurred because the object noticeably changes po- ⌬ sity or pressure could be deduced. Snap- spectively) and a sufficiently high timing sition during exposure. From position change x shots were taken at different delays be- accuracy between each. Because the oscil- (which can be assessed from the blurring) and speed of the motorcyclist, the camera exposure tween the first spark that triggered the lation cycle of sound waves is of the order ⌬ time can be readily determined: exp = x/ (if the sound wave and the second that emitted a of a millisecond or somewhat less, a flash camera is assumed to be stationary for the sake of light flash. The temporal evolution of the of microsecond duration with microsec- simplicity).This same concept forms the basis for density oscillations in the acoustic wave ond timing accuracy allowed Töpler to evaluation of the subfemtosecond x-ray pulse du- could be reconstructed from the series of take freeze-frame shots of the waves at ration from the x-ray photoelectron spectra freeze-frame shots. precise instants to reconstruct their oscil- shown in Fig. 6. The success of Töpler’s pioneering ex- lations. In principle, the same technique periment relied on a sufficiently short du- might ultimately prove suitable for prob- May 2002 I Optics & Photonics News 63 ATTOSECOND SPECTROSCOPY 1400 3.0 ing the electromagnetic field of a light More than a hundred years later, im- 1200 =/2 wave provided that a sampling apparatus, plementation of Töpler’s concepts in con- 2.5 1000 =0 with a level of resolution measured in at- junction with ultrashort laser pulses paved 2.0 800 toseconds, or in other words, a trillion the way for much higher time resolutions 1.5 times higher than that of the device used 600 and opened the door for the study of a by Töpler, were available. More than a wide range of microscopic dynamics. Ul- 400 1.0 century after Töpler’s experiment, as can trashort light pulses of sufficient energy, 200 0.5 be seen in the “After Image” photo that ap- delivered by mode-locked lasers,4 can be 0 0.0 Temporal Intensity (arb. u.) Temporal -4 -3 -2 -1 0 1 2 3 4 5 pears on p. 80 of this issue of OPN, such a used to induce measurable (either tran- On-axis Electric Field (arb. u.) Time (fs) sampling apparatus does indeed exist. The sient or permanent) changes in the elec- photograph shows how x-ray photoelec- tronic or nuclear structure of matter. Mea- EL(t)=A(t) cos ( Lt+ ) tron spectra produced in the presence of a suring the characteristics of photons or strong light field exhibit modulations that electrons that exit the sample after irradia- Figure 3. Calculated far-field, near-axis temporal trace the electric field oscillations in a visi- tion by a delayed replica of the same pulse, intensity profile of a soft-x-ray pulse (solid curves) ble light wave. The attosecond sampling one can record snapshots of the evolving that emerges from few-cycles-driven high-harmon- system used to take this measurement of- ic generation.The x-ray photons are selected with- microscopic structure of the investigated in a 5-eV spectral range near 90 eV and are pro- fers an unparalleled level of temporal reso- sample. From the snapshots taken at dif- duced in a 3-mm-long 200-mbar neon-gas source lution for studies of the fundamental dy- ferent arrival times of the probe pulse, one 3 by a 7-fs (full width at half-maximum), 750-nm namics of matter. Snapshots taken with can retrieve the temporal evolution of the Gaussian laser pulse with an on-axis peak intensity attosecond exposure allow us to capture microscopic dynamics. The concept can of 9 x 1014 W/cm2. For the electric field of the driv- the motion of electrons in atoms, for the be implemented with an all-optical sam- 2 2 ing laser pulse, E L(t) exp(-t / L ) cos( 0 t+ ) was first time to my knowledge, by means of pling system, as illustrated in Fig. 1. assumed at the input of the gas source, where , L attosecond spectroscopy. What can we sample with an all-optical , and represent the pulse duration, angular 0 sampling system? The answer depends on carrier frequency, and absolute phase of the few- cycles pump pulse. The dashed curves show the Time-resolved spectroscopy the response time of the light-induced ex- on-axis electric field of the laser pulse that exits In his pioneering experiment, Töpler in- citations, relaxations, or displacements of the interaction region for an initial absolute phase troduced several new concepts of far- electrons and/or nuclei in the illuminated of = 0 (cosine pulse, red curve)and = /2 (sine reaching importance that form the basis of sample. The response time can be fast or pulse, blue curve), and the solid red and blue modern time-resolved spectroscopy (also slow depending on the duration of the curves that depict the calculated x-ray intensities, known as pump–probe spectroscopy). pump and the probe light. An important in a frame that moves at the phase velocity of the First, to ensure synchronization, he used specific example for the fast response lim- laser pulse. Efficient generation of isolated subfem- it is light-field-induced electron displace- tosecond x-ray pulses under the above experi- the same event to trigger the process under ment in a transparent (insulating) crystal. mental conditions is predicted for ≈ 0 only, indi- investigation and to produce the flash that cating the importance of stabilization and control probed it. Second, he introduced a variable The induced dipole moment and macro- of the absolute phase. delay between the triggering event (pump scopic polarization associated with this displacement tend to be a slightly nonlin- ear function of the instantaneous light field, resulting in the emission of photons at the second harmonic of the incident light waves. Measuring the second-har- X-ray pulse monic photon flux as a function of delay Electrons, between two identical replicas of the inci- Photons dent light pulse yields a nonlinear auto- correlation function that provides insight Beam Detector splitter into the temporal structure of the light Visible light wave Interaction bursts.
Details
-
File Typepdf
-
Upload Time-
-
Content LanguagesEnglish
-
Upload UserAnonymous/Not logged-in
-
File Pages7 Page
-
File Size-