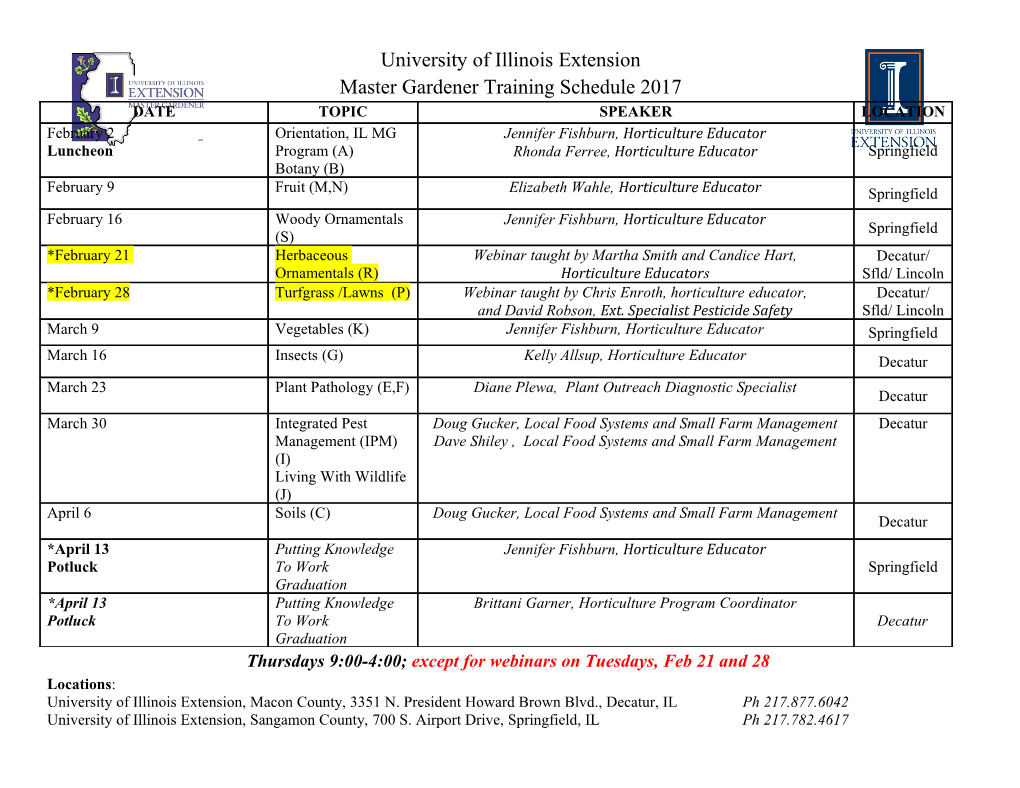
Geophysical Research Letters RESEARCH LETTER Wind-driven freshwater buildup and release in the Beaufort 10.1002/2015GL065957 Gyre constrained by mesoscale eddies Key Points: 1 1 • Wind-driven Beaufort Gyre dynamics Georgy E. Manucharyan and Michael A. Spall is simulated within an eddy-resolving model 1Department of Physical Oceanography, Woods Hole Oceanographic Institution, Woods Hole, Massachusetts, USA • Mesoscale eddies play a key role in constraining variations in freshwater content Recently, the Beaufort Gyre has accumulated over 20,000 km3 of freshwater in response to • The Beaufort Gyre is currently at its Abstract highest sensitivity to wind stress strong anticyclonic atmospheric winds that have prevailed over the gyre for almost two decades. Here we forcing explore key physical processes affecting the accumulation and release of freshwater within an idealized eddy-resolving model of the Beaufort Gyre. We demonstrate that a realistic halocline can be achieved when Correspondence to: its deepening tendency due to Ekman pumping is counteracted by the cumulative action of mesoscale G. E. Manucharyan, eddies. Based on this balance, we derive analytical scalings for the depth of the halocline and its spin-up [email protected] time scale and emphasize their explicit dependence on eddy dynamics. Our study further suggests that the Beaufort Gyre is currently in a state of high sensitivity to atmospheric winds. However, an intensification of Citation: surface stress would inevitably lead to a saturation of the freshwater content—a constraint inherently set Manucharyan, G. E., and M. A. Spall by the intricacies of the mesoscale eddy dynamics. (2015), Wind-driven freshwater buildup and release in the Beaufort Gyre constrained by mesoscale eddies, Geophys. Res. Lett., 42, doi:10.1002/2015GL065957. 1. Introduction The Arctic Ocean is an essential part of our changing climate system [Moritz et al., 2002]. Because it is strongly Received 27 AUG 2015 affected by atmospheric dynamics, radiative processes, and is interconnected with both the Pacific and Accepted 1 OCT 2015 Atlantic Oceans, the Arctic maintains a complex circulation balancing heat and salt budgets. Time depen- Accepted article online 5 OCT 2015 dence in forcing can lead to a strong response in sea ice cover [Eisenman and Wettlaufer, 2009; Boé et al., 2009; Schweiger et al., 2011; Krishfield et al., 2014], circulation patterns [Dickson et al., 2000; Morison et al., 2012; McPhee, 2013], CO2 uptake [Caietal., 2010], and biomass production [Tremblay et al., 2011; McLaughlin and Carmack, 2010; Li et al., 2009]. Here we bring the reader’s attention to the freshwater content that has been increasing primarily in the Beaufort Gyre [Rabe et al., 2011, 2014; Haine et al., 2015]—a major anticyclonic cir- culation in the upper 300 m of the Canada Basin in the Arctic Ocean [Aagaard and Carmack, 1989; Proshutinsky et al., 2002]. The surface freshwater provides a stratification cap preventing deep but warm Atlantic waters from reaching the surface and melting the sea ice. Furthermore, excessive freshwater can propagate from the Arctic to the North Atlantic Ocean and potentially affect the formation of deep water masses—an important component of the Meridional Overturning Circulation. It has also been hypothesized that freshwater from the Arctic might have contributed to Great Salinity Anomalies [Dickson et al., 1988; Belkin et al., 1998; Belkin, 2004] with poten- tial consequences for the global climate [Häkkinen, 1999; Zhang and Vallis, 2006]. Nonetheless, a quantitative understanding of the gyre’s freshwater accumulation and release is not well developed. Observational and modeling studies identify the importance of primarily anticyclonic (clockwise) surface stress (Figure 1) as a forcing for the gyre [Proshutinsky and Johnson, 1997; Proshutinsky et al., 2002, 2009; Timmermans et al., 2011; Watanabe, 2013] which can change to weakly cyclonic regimes and affect the gyre circulation [Proshutinsky and Johnson, 1997; Proshutinsky et al., 2002]. The converging Ekman flow associated with the anticyclonic atmospheric stress advects relatively fresh surface waters from the boundaries and pumps them into the interior of the gyre, thus deepening the halocline (a region of sharp vertical gradient in salinity) and increasing the freshwater content (FWC) of the gyre. However, the accumulation of freshwa- ter under steady surface stress forcing cannot last indefinitely, and a steady state gyre can only be achieved if there is a process opposing this freshwater pumping. Here we point to the cumulative action of mesoscale eddies as a major mechanism that counteracts the halo- ©2015. American Geophysical Union. cline deepening due to Ekman pumping. Because the halocline is shallower near the coastal boundaries and All Rights Reserved. deepens toward the interior, the gyre not only holds FWC but also contains a large amount of gravitational MANUCHARYAN AND SPALL EDDY CONSTRAINED BEAUFORT GYRE 1 Geophysical Research Letters 10.1002/2015GL065957 potential energy. Analogous to many other regions of the World Ocean, this potential energy can be released through the process of baroclinic instability accompanied by the gen- eration of mesoscale eddies [Pedlosky, 1982; Vallis, 2006]. Cumulatively, these eddies act to flatten the halocline [Marshall and Radko, 2003; Karsten et al., 2002] thus constraining its deep- ening and setting its bulk properties [Marshall et al., 2002]. Observations in the Arctic Ocean reveal that it is populated with mesoscale eddies [Manley and Hunkins, 1985; Timmermans et al., 2008; Dmitrenko et al., 2008; Watanabe, 2011; Zhao et al., 2014] with studies suggesting that the origins of such eddies are the instabili- ties of the oceanic fronts [Manucharyan and Timmermans, 2013] and boundary currents [Watanabe, 2013; Spall et al., 2008; Spall, 2013]—all associated with the release of the accumulated gravi- Figure 1. Representative conditions of the Arctic Ocean. Colors: tational potential energy. Nonetheless, freshwater content averaged from a compilation of in situ observations despite mesoscale eddies being ubiq- over the period of 2000–2009. Black contours: sea level pressure obtained uitously observed in the Beaufort Gyre, from National Centers for Environmental Prediction reanalysis for the same period. Arrows: geostrophic winds calculated based on the sea level their cumulative influence on its fresh- pressure. Note the presence of anticyclonic atmospheric winds water balance has not been clearly surrounding a region of maximum freshwater content. Figure courtesy established. of A. Proshutinsky. Here we investigate the major physical processes that govern the accumula- tion and release of freshwater in the Beaufort Gyre. We illustrate our discussions using an observationally consistent conceptual model of the gyre that explicitly resolves mesoscale eddies. 2. Beaufort Gyre Model 2.1. Formulation We focus on the internal gyre dynamics and apply an idealized modeling approach combined with analyti- cal investigations. We model the Beaufort Gyre in a closed circular basin with a radius of 600 km representing an area with the observed maximum in Arctic FWC (Figure 1). Because the water mass exchanges with the Pacific and Atlantic Oceans primarily occur through topographically trapped boundary currents [Rudels, 2012; Aksenov et al., 2011], we parameterize them by restoring salinity at the boundaries to a representative ver- tical profile. This near-coastal salinity distribution is set by a complex interplay between strongly variable inflows of both fresh and salty waters affected by intermittent wind-driven coastal upwelling [Pickart et al., 2013]—processes which we do not attempt to model here. Instead, consistent with observations, we impose a typical salinity profile at the boundaries which consists of a fresher upper layer of about 50 m thickness (salinity of 29) and salty waters beneath (bottom salinity 34). While there are indications of decadal variability of river outflows [Peterson et al., 2002] and changes in Atlantic and Pacific water inflow [Bourgain and Gascard, 2012], we consider these processes (as well as changes in sea ice [Krishfield et al., 2014]) to be secondary to the basic wind-driven dynamics of the gyre. We note, however, that these processes can significantly affect the temporal and spacial variability of the gyre. Nonetheless, for simplicity, we keep the coastal salinity profile constant in time and allow the interior of the gyre to naturally draw a necessary amount of fresh/salty waters from its boundaries as is required to satisfy its wind-driven adjustment. MANUCHARYAN AND SPALL EDDY CONSTRAINED BEAUFORT GYRE 2 Geophysical Research Letters 10.1002/2015GL065957 Figure 2. (a) Instantaneous field of halocline depth (in meters) for the equilibrated gyre state numerically simulated for a . −2 reference surface stress ( 0 = 0 015 Nm ). Note the presence of radial gradients in halocline depth and a pronounced eddy field. (b) Azimuthally averaged salinity distribution (in color). Overimposed is a schematic view of the gyre features: wind-driven Ekman pumping at the surface, opposing eddy-driven circulation (arrows), and the resulting halocline layer that is deeper in the interior. Note that the model domain extends to 900 m depth, but here salinity field is shown only down to 450 m below which the isopycnals are almost flat. We force the gyre via the idealized anticyclonic surface stress with the fluid dynamical and salt transport pro- cesses simulated in an eddy-resolving configuration
Details
-
File Typepdf
-
Upload Time-
-
Content LanguagesEnglish
-
Upload UserAnonymous/Not logged-in
-
File Pages10 Page
-
File Size-