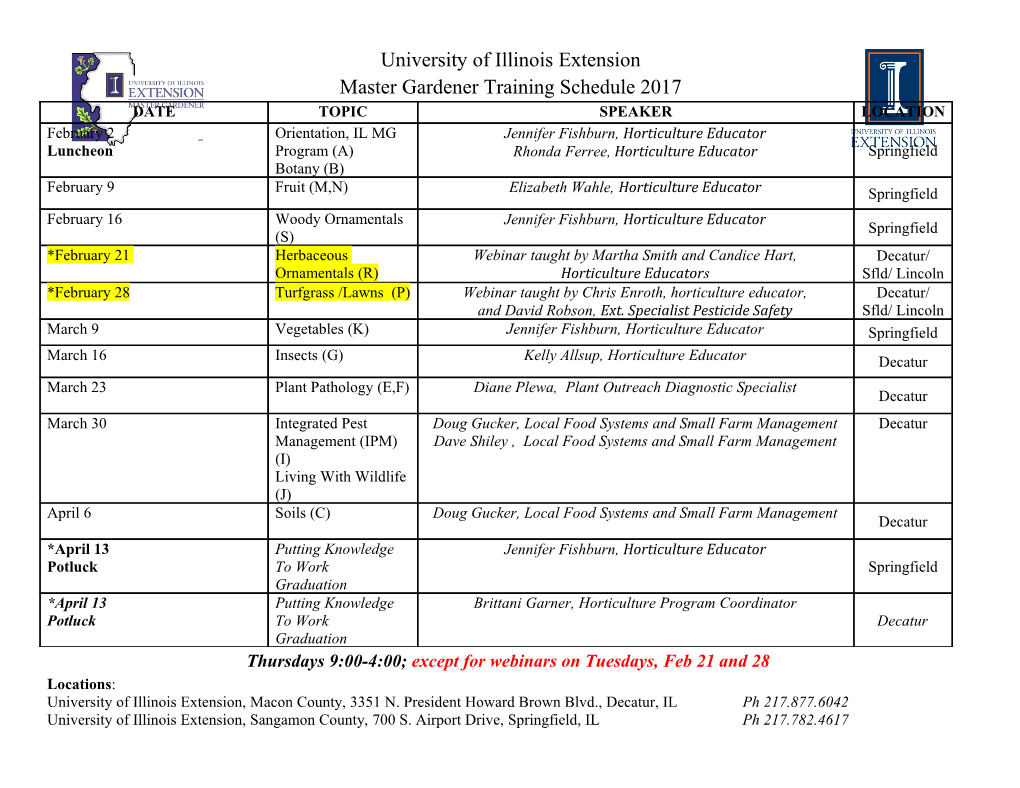
Progress in Oceanography Progress in Oceanography 75 (2007) 70–114 www.elsevier.com/locate/pocean Interactions between the Indonesian Throughflow and circulations in the Indian and Pacific Oceans Julian P. McCreary a,*, Toru Miyama b, Ryo Furue a, Tommy Jensen a, Hyoun-Woo Kang a,1, Bohyun Bang a, Tangdong Qu a a International Pacific Research Center, University of Hawaii, Honolulu, HI, United States b Frontier Research System for Global Change, Yokohama, Japan Received 25 October 2006; received in revised form 16 April 2007; accepted 25 May 2007 Available online 6 July 2007 Abstract Circulations associated with the Indonesian Throughflow (IT), particularly concerning subsurface currents in the Pacific 1 Ocean, are studied using three types of models: a linear, continuously stratified (LCS) model and a nonlinear, 4 2-layer model (LOM), both confined to the Indo-Pacific basin; and a global, ocean general circulation model (COCO). Solutions are wind forced, and obtained with both open and closed Indonesian passages. Layers 1–4 of LOM correspond to near- surface, thermocline, subthermocline (thermostad), and upper-intermediate (AAIW) water, respectively, and analogous layers are defined for COCO. The three models share a common dynamics. When the Indonesian passages are abruptly opened, barotropic and baroclinic waves radiate into the interiors of both oceans. The steady-state, barotropic flow field from the difference (open À closed) solution is an anticlockwise circulation around the perimeter of the southern Indian Ocean, with its merid- ional branches confined to the western boundaries of both oceans. In contrast, steady-state, baroclinic flows extend into the interiors of both basins, a consequence of damping of baroclinic waves by diapycnal processes (internal diffusion, upwelling and subduction, and convective overturning). Deep IT-associated currents are the subsurface parts of these baroclinic flows. In the Pacific, they tend to be directed eastward and poleward, extend throughout the basin, and are closed by upwelling in the eastern ocean and Subpolar Gyre. Smaller-scale aspects of their structure vary significantly among the models, depending on the nature of their diapycnal mixing. At the exit to the Indonesian Seas, the IT is highly surface trapped in all the models, with a prominent, deep core in the LCS model and in LOM. The separation into two cores is due to near-equatorial, eastward-flowing, subsurface currents in the Pacific Ocean, which drain layer 2 and layer 3 waters from the western ocean to supply water for the upwelling regions in the eastern ocean; indeed, depending on the strength and parameterization of vertical diffusion in the Pacific interior, the draining can be strong enough that layer 3 water flows from the Indian to Pacific Ocean. The IT in COCO lacks a signif- icant deep core, likely because the model’s coarse bottom topography has no throughflow passage below 1000 m. Consis- tent with observations, water in the near-surface (deep) core comes mostly from the northern (southern) hemisphere, a * Corresponding author. Address: IPRC/SOEST, University of Hawaii, POST Bldg. 401, 1680 East West Road, Honolulu, HI 96822, United States. Tel.: +1 808 956 2216; fax: +1 808 956 9425. E-mail address: [email protected] (J.P. McCreary). 1 Present address: Coastal and Harbor Engineering Laboratory, Korea Ocean Research and Development Institute, Ansan, Republic of Korea. 0079-6611/$ - see front matter Ó 2007 Elsevier Ltd. All rights reserved. doi:10.1016/j.pocean.2007.05.004 J.P. McCreary et al. / Progress in Oceanography 75 (2007) 70–114 71 consequence of the wind-driven circulation in the tropical North Pacific being mostly confined to the upper ocean; as a result, it causes the near-surface current along the New Guinea coast to retroflect eastward, but has little impact on the deeper New Guinea undercurrent. In the South Pacific, the IT-associated flow into the basin is spread roughly uniformly throughout all four layers, a con- sequence of downwelling processes in the Indian Ocean. The inflow first circulates around the Subtropical Gyre, and then bends northward at the Australian coast to flow to the equator within the western boundary currents. To allow for this additional, northward transport, the bifurcation latitude of the South Equatorial Current shifts southward when the Indo- nesian passages are open. The shift is greater at depth (layers 3 and 4), changing from about 14°S when the passages are closed to 19°S when they are open and, hence, accounting for the northward-flowing Great Barrier Reef Undercurrent in that latitude range. After flowing along the New Guinea coast, most waters in layers 1–3 bend offshore to join the North Equatorial Coun- tercurrent, Equatorial Undercurrent, and southern Tsuchiya Jet, respectively, thereby ensuring that northern hemisphere waters contribute significantly to the IT. In contrast, much of the layer 4 water directly exits the basin via the IT, but some also flows into the subpolar North Pacific. Except for the direct layer 4 outflow, all other IT-associated waters circulate about the North Pacific before they finally enter the Indonesian Seas via the Mindanao Current. Ó 2007 Elsevier Ltd. All rights reserved. Keywords: Indonesian Throughflow; Pacific Ocean; Indian Ocean; Antarctic Intermediate Water; Thermostad water; Great Barrier Reef Undercurrent; Tsuchiya Jets 1. Introduction 1.1. Observations The Indonesian Seas (Fig. 1) allow primarily warm and fresh, near-surface waters to flow from the Pacific into the Indian Ocean. Estimates of the mean transport of the Indonesian Throughflow (IT) range from 2 to more than 20 Sv (Sv = 106 m3 sÀ1), the large variability a consequence of the lack of direct observations and the IT’s large seasonal-to-interannual variability (Wyrtki, 1961; Gordon, 1986; Godfrey et al., 1993; Meyers 10N MC Sulu Sea NEC S. China Sea NECC Sulawesi Sea Halmahera Sea Karimata Str. Maluku Str. EUC 0 Borneo Halmahera Sulawesi NGCUC New Guinea Java Sea Makassar Str. Banda Sea Flores Sea Ombai Str. 10S Torres Str. IT Lombok Str. Timor Pass. Coral Sea Australia 110E 120E 130E 140E 150E Fig. 1. Map of the Indonesian Seas, as well as nearby regions in the Pacific and Indian Oceans with areas shallower than 200 m lightly shaded. Solid arrows indicate major surface currents, including the Mindanao Current (MC), North Equatorial Current (NEC), and North Equatorial Countercurrent (NECC), as well as the Indonesian Throughflow (IT). The dashed arrow indicates the New Guinea Coastal Undercurrent (NGCUC) and Equatorial Undercurrent (EUC). 72 J.P. McCreary et al. / Progress in Oceanography 75 (2007) 70–114 et al., 1995; Schiller et al., 1998; Potemra, 1999; Lebedev and Yaremchuk, 2000). More recent estimates, how- ever, favor a value near the middle of this range (Gordon et al., 1999; Vranes et al., 2002; Susanto and Gor- don, 2005). Most of the transport exits in the upper ocean above 400 m and comes from the North Pacific (Gordon and Fine, 1996; Gordon et al., 1999; Ffield et al., 2000; Gordon, 2005); there is also a deep relative maximum, consisting primarily of water from the South Pacific (Molcard et al., 1996; Talley and Sprintall, 2005; Gordon, 2005). Since the North Pacific is essentially closed, source waters for the IT necessarily originate from the South Pacific, specifically from the intrusion of Antarctic Circumpolar (ACC) water into the basin (McCartney, 1977, 1982; Talley et al., 1996; Gordon and Fine, 1996). Much of it is entrained into the Subtropical Gyre (STG) in the far-eastern South Pacific as an upper part of Antarctic Intermediate Water (AAIW), and subse- quently advects around the gyre as a tongue of low-salinity and high-oxygen water within the South Equato- rial Current (SEC). The SEC bifurcates at the Australian coast, with water in its northern part flowing equatorward and in its southern part returning to midlatitudes. The deep equatorward branch flows continuously along the Austra- lian coast, eventually joining the deep part of the New Guinea Coastal Undercurrent (NGCUC) that extends to a depth of 900 m or more (Lindstrom et al., 1987, 1990; Tsuchiya, 1991; Qu and Lindstrom, 2002). There- after, AAIW spreads throughout the tropics (Reid, 1965, 1997; Talley, 1999; Zenk et al., 2005). There is evi- dence that AAIW also flows into the North Pacific (Rochford, 1960; Reid, 1965; Tsuchiya, 1991; Fine et al., 1994), as part of the northward-flowing Mindanao Undercurrent (Hu et al., 1991; Qu et al., 1998). Recent observations indicate that AAIW does not extend as a distinct water mass much farther north than 15°N in the western North Pacific (Qu et al., 1999; Qu and Lindstrom, 2004); farther north, it loses its distinguishing salinity minimum, and its fate remains unknown. Another prominent, subthermocline water mass is thermostad water, located just beneath the near-equato- rial thermocline. Like AAIW, it also originates in the South Pacific, either from water subducted north of New Zealand (Tsuchiya, 1991; Roemmich and Cornuelle, 1992) or from Subantarctic Mode Water (SAMW) gen- erated in the eastern South Pacific (Toggweiler et al., 1991). In either case, the subducted water may still arise from the ACC, via near-surface northward flow that is modified by surface fluxes before it subducts; it could also originate within the Pacific via southward flow of near-surface water from the tropics, forming an over- turning cell within the basin that is somewhat broader and deeper than the South Pacific Subtropical Cell (McCreary and Lu, 2001). 1.2. Models Models have contributed significantly toward understanding impacts of the IT-associated, Interocean Cir- culations (IOCs) in both the Indian and Pacific Oceans (see Godfrey, 1996, for a review). The models vary in their domain size, including models confined to the Indian Ocean (McCreary and Kundu, 1987; Godfrey and Weaver, 1991; Hughes et al., 1992) and Pacific Ocean (Lu et al., 1998; McCreary and Lu, 2001; McCreary et al., 2002) in which the IT is externally prescribed, as well as global models in which it is generated internally (Miyama et al., 1995; Verschell et al., 1995; Shriver and Hurlburt, 1997; Murtugudde et al., 1998; Rodgers et al., 1999; Schneider, 1998; Lee et al., 2002).
Details
-
File Typepdf
-
Upload Time-
-
Content LanguagesEnglish
-
Upload UserAnonymous/Not logged-in
-
File Pages45 Page
-
File Size-