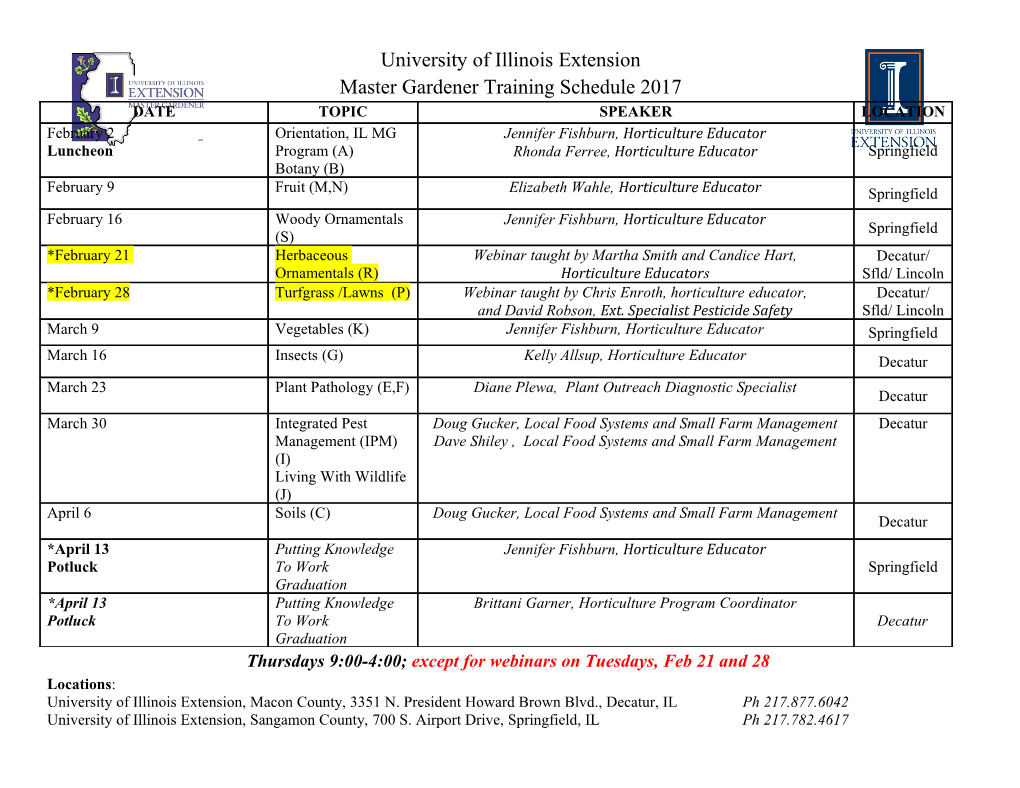
2 Spectroscopy 32(4) April 2017 www.spectroscopyonline.com Pump–Probe Microscopy: Theory, Instrumentation, and Applications Excited-state dynamics provides an intrinsic molecular contrast of samples examined. These dynam- ics can be monitored by pump–probe spectroscopy, which measures the change in transmission of a probe beam induced by a pump beam. With superior detection sensitivity, chemical specificity, and spatial–temporal resolution, pump–probe microscopy is an emerging tool for functional imag- ing of nonfluorescent chromophores and nanomaterials. This article reviews the basic principle, instrumentation strategy, data analysis methods, and applications of pump–probe microscopy. A brief outlook is provided. Pu-Ting Dong and Ji-Xin Cheng s a pioneer of femtochemistry, Nobel laureate Ahmed copy to measure fluorescence lifetime (13). In 2007, the Warren Hassan Zewail (1–3)recorded the snapshots of chemi- group reported pump–probe imaging with a high-frequency A cal reactions with sub-angstrom resolution through modulation scheme (14). Their work demonstrated the fea- an ultrafast femtosecond transient absorption (TA) technique. sibility of imaging melanin by using two-color two-photon In a transient absorption experiment, a laser pulse pumps a absorption (TPA) or excited state absorption (ESA) processes. molecule into an excited state. The excited state itself exhibits Since then, extensive research has been conducted by har- relaxation dynamics on the femtosecond or picosecond tim- nessing the merits of pump–probe microscopy. A majority of escale. A second laser pulse then probes the population in the the research focused on nonfluorescent chromophores such excited state at different temporal delays with respect to the as hemoglobin and cytochromes, which absorb light but do excitation. This analysis method reveals the dynamics of the not emit fluorescence efficiently (15). Fu and colleagues used excited state and is termed as pump–probe spectroscopy. two-color absorption to measure the degree of oxygenation Pump–probe microscopy, also known as transient absorp- based on the different decay constants of deoxyhemoglobin tion microscopy, is an emerging nonlinear optical imaging and oxyhemoglobin (16). Pump–probe microscopy can effi- technique that probes the excited state dynamics, which is re- ciently discern hemoglobin and melanin, the two major ab- lated to the third-order nonlinearity (3,4). Pump–probe mi- sorbers in a biological tissue. Based on their signatures from croscopy is an attractive spectroscopic imaging technique with the time-resolved curves, hemoglobin shows a purely positive the following advantages: First, it is nondestructive to cells and response because of excited state absorption, whereas melanin tissues and can be performed without tissue removal (5). Thus, (eumelanin and pheomelanin) demonstrate a negative (ground it can be used as a repeatable diagnostic tool. Second, it is a state bleaching) signal when the pump beam and probe beam label-free technique and doesn’t need an exogenous target (4). spatially and temporally overlap (5). In addition, pump–probe Third, as a nonlinear optical technique, pump–probe micros- microscopy enables the discrimination of melanomas by de- copy can image endogenous pigments with three dimensional termining the ratio between eumelanin and pheomelanin. (3-D) spatial resolution (6). Fourth, unlike linear absorption, Melanin play an important role in skin and hair pigmentation which suffers from scattering in a tissue sample, the pump– and melanomas (17). Without external staining, pump–probe probe technique only measures absorption at the focal plane, imaging yielded novel insight into the differentiation of eu- which offers optical sectioning capability (6). Fifth, compared melanin and pheomelanin among thin biopsy slices and has to scattering measurements, this absorption-based method been used to probe the metastatic potential of melanocytic has a weaker dependence on the particle and thus is highly cutaneous melanomas (16). Besides applications to pigments sensitive to nanoscale subjects (8–11). Sixth, pump–probe in biological tissue, pump–probe microscopy has also been microscopy with near-infrared laser pulses permits biological applied to distinguish various kinds of pigments in arts based applications with an enhanced penetration depth and a lower on their decay differences (18–21). level of tissue damage (12). Another significant application of pump–probe microscopy In 1990s, Dong and coworkers used pump–probe micros- is for characterization of single nanostructures including gold www.spectroscopyonline.com April 2017 Spectroscopy 32(4) 3 nanorods (22)and single-wall nanotubes (SWNTs) (23–26). Specifically, Jung and coworkers for the first time deployed the phase of the pump–probe signal as a contrast to distinguish semiconducting Second excited state carbon nanotubes from metallic ones (25). Tong and colleagues further used this contrast for imaging semiconduct- ing and metallic nanotubes in living Pump Probe cells (26). By tuning the excitation wave- First excited state length, which is resonant with the lowest Vibrational state electronic transition in SWNTs, Huang and colleagues exploited the band-edge relaxation dynamics in isolated and bundled SWNTs (23). Through as- Excited state absorption Stimulated emssion Ground state depletion sembling SWNTs with CdS, Robel and Ground state colleagues demonstrated the charge- transfer interaction between photoex- Figure 1: Three major processes in a pump–probe experiment: (a) Excited state absorption, (b) cited CdS nanoparticles and SWNTs by stimulated emission, and (c) ground-state depletion. For ground-state depletion, the number of transient absorption (24). the molecules in the ground state is decreased upon photoexcitation, consequently increasing In this review, we summarize the con- the transmission of the probe pulse. For stimulated emission, photons in its excited state can trast mechanisms and instrumentation be stimulated down to the ground state by an incident light field, thus leading to an increase of strategies of pump–probe microscopy transmitted light intensity on the detector. In the case of excited-state absorption, the probe photons and highlight some of these significant are absorbed by the excited molecules, promoting them to the higher energy levels. applications. Because of space limita- tions, we could not cover the entire lit- erature and would recommend to the readers other excellent articles in this Pump pulse train Motorized delay line field (27–32). tau Pump–Probe Theory In a typical pump–probe measurement, Probe Pump the pump-induced intensity change of the probe is measured by a lock-in Scanning mirrors AOM amplifier referenced to the modulated Modulation frequency Transferred modulation pump pulse. Then this change is nor- tau Loss Pump pulse train Objective malized by the probe beam intensity Delay stack Sample Δ Gain Condenser to generate Ipr/Ipr (33). To express this Lock-in amplier Photodiode process at molecular level, we define the Ref Out In absorption coefficient for an electronic Filter transition between level “i” and level “j” as Figure 2: Schematic illustration of pump probe microscopy. αij(ω) = σij(ω) (Ni– Nj) [1] where σij(ω) is the cross section from electronic state i to j, and Ni and Nj are beam: observed: When the probe pulse is res- the populations of the initial and final onant with i→j transitions (i ≠ 0), then ΔIpr states, respectively. Conventionally, α is =−∑αij(ω)ΔNj d [2] the probe pulse is absorbed by the mol- I i,j positive for absorption and negative for pr ecule, reducing the transmission of the gain (33). where d is the sample thickness. The probe pulse. This negative ΔIpr/Ipr signal The pump pulse acts on the sample expression is derived from the Lambert- change is therefore called excited state by changing the energy level popula- Beer relation within the small signal ap- absorption (ESA). When the probe pulse tion, N→N + ΔN. As a consequence, the proximation. The “j” term describes all is resonant with 0→j transmission, the population of excited states will increase possible excited states (33). probe transmission is enhanced upon at the expense of that of the ground state. Depending on the probe energy, three pump excitation. This positive ΔIpr/Ipr Such change is measured by the probe effects on the transmitted pulse can be phenomenon is called ground-state de- 4 Spectroscopy 32(4) April 2017 www.spectroscopyonline.com pletion (GSD). When the lowest excited state is dipole-coupled to the ground (a) Pump-probe AFM image (b) 1.6 1.6 Pump ) state and the probe pulse is resonant ) -1 1.2 1.2 Probe with the transition, stimulated emission cm -1 0.8 0.8 (SE) occurs. An increased transmission M 5 is observed in a SE process. (10 0.4 0.4 These three major processes are illus- Fluorescence (a.u. 0.0 0.0 trated in Figure 1. A detailed description 500 600 700 800 Wavelength (nm) is provided in the following sections. STAM 100 30 ) Pump-probe STAM (x2.5) ) -6 20 Excited-State Absorption Mean molecule 50 No. in focus Excited-state absorption (ESA) is a 0 1 2 3 4 10 0.4 process where the probe photons are ∂∂P/P (10 0.2 285 nm attenuated by excited states as shown 0 280 nm 0.0 Signal intensity (a.u. 0 0.0 0.5 1.0 1.5 0.0 0.1 0.2 in Figure 1. Since the 1970s, picosec- Position (μm) 0 2 4 6 8 10 Concentration (μM) ond laser–based ESA measurements have been extensively used to measure Figure 3: Pump probe microscopy with subdiffraction spatial resolution and single-molecule detection ground and excited-state dynamics sensitivity. (a) Subdiffraction-limited imaging of graphite nanoplatelets. Image from conventional (34,35). Compared to two-photon ab- transient absorption microscopy (top left) and AFM image of graphite nanoplatelets (top right). Image sorption, which goes through a virtual from saturation transient absorption microscopy (bottom left) and intensity profiles along the lines intermediate state, excited-state absorp- indicated by the dashed lines in pump–probe image and STAM image (bottom right).
Details
-
File Typepdf
-
Upload Time-
-
Content LanguagesEnglish
-
Upload UserAnonymous/Not logged-in
-
File Pages10 Page
-
File Size-