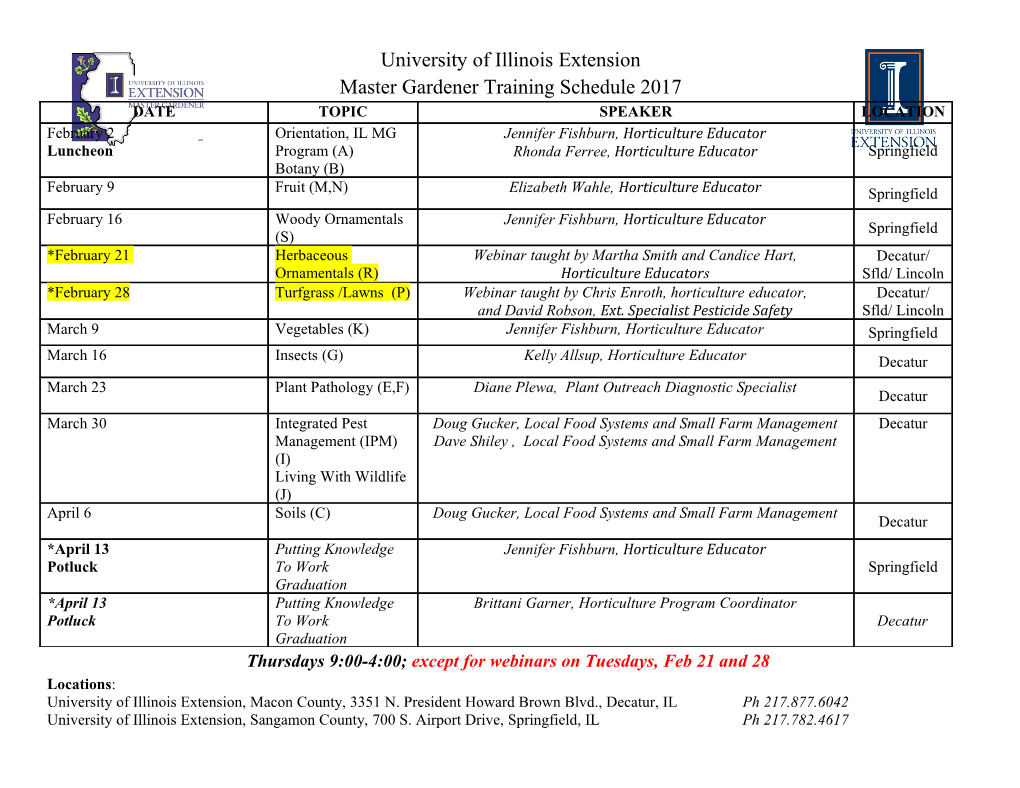
Cognitive Computation (2019) 11:6447777–655 https://doi.org/10.1007/s12559-019-09658-8 Improving the Recall Performance of a Brain Mimetic Microcircuit Model Vassilis Cutsuridis1 Received: 8 February 2019 /Accepted: 30 May 2019 / Published online: 13 June 2019 # Springer Science+Business Media, LLC, part of Springer Nature 2019 Abstract The recall performance of a well-established canonical microcircuit model of the hippocampus, a region of the mammalian brain that acts as a short-term memory, was systematically evaluated. All model cells were simplified compartmental models with complex ion channel dynamics. In addition to excitatory cells (pyramidal cells), four types of inhibitory cells were present: axo- axonic (axonic inhibition), basket (somatic inhibition), bistratified cells (proximal dendritic inhibition) and oriens lacunosum- moleculare (distal dendritic inhibition) cells. All cells’ firing was timed to an external theta rhythm paced into the model by external reciprocally oscillating inhibitory inputs originating from the medial septum. Excitatory input to the model originated from the region CA3 of the hippocampus and provided context and timing information for retrieval of previously stored memory patterns. Model mean recall quality was tested as the number of stored memory patterns was increased against selectively modulated feedforward and feedback excitatory and inhibitory pathways. From all modulated pathways, simulations showed recall performance was best when feedforward inhibition from bistratified cells to pyramidal cell dendrites is dynamically increased as stored memory patterns is increased with or without increased pyramidal cell feedback excitation to bistratified cells. The study furthers our understanding of how memories are retrieved by a brain microcircuit. The findings provide fundamental insights into the inner workings of learning and memory in the brain, which may lead to potential strategies for treatments in memory-related disorders. Keywords Hippocampus . Inhibition . Excitation . Bistratified cell . Schaffer collateral . Medial septum Introduction a noisy or partial version of a previously stored patterns was presented to the network. However, these devices were not We are continually bombarded with sensory information, very flexible. They had to be told when to store a pattern some of which we remember and some of which sparks the and when they were supposed to recall a memory. The types recall of old memories. Some aspects of how the brain may of information they could store were quite limited. store and recall information were captured in mathematical In 2010, a much more flexible model was introduced that artificial neural network models known as associative memo- controlled for itself the storage and recall of patterns of infor- ries, which were first developed over 50 years ago [35, 37]. mation arriving at unpredictable rates [10]. The model was These models worked by storing patterns of information via based upon the many details we knew of the neuronal hippo- changes in the strengths of connections between simple com- campal circuit, a part of the mammalian brain that acts as a puting units that mimicked the operation of neurons in the short-term memory. The model explored the functional roles brain in a very simple way. Old memories were recalled when of somatic, axonic and dendritic inhibition in the encoding and retrieval of memories in region CA1 of the hippocampus. It Electronic supplementary material The online version of this article showed how theta modulated inhibition separated encoding (https://doi.org/10.1007/s12559-019-09658-8) contains supplementary and retrieval of memories in the hippocampus into two func- material, which is available to authorized users. tionally independent processes. It predicted that somatic inhi- bition allowed generation of dendritic calcium spikes that pro- * Vassilis Cutsuridis moted synaptic long-term plasticity (LTP), while minimizing [email protected]; [email protected] cell output. Proximal dendritic inhibition controlled both cell 1 School of Computer Science, Brayford Pool, University of Lincoln, output and suppressed dendritic calcium spikes, thus Lincoln LN6 7TS, UK preventing LTP, whereas distal dendritic inhibition removed 645 Cogn Comput (2019) 11:644–655 interference from spurious memories during recall. The mean types reported in the literature, which were extensively validated recall quality of the model was tested as function of memory against experimental data in [27–30]. The complete mathemati- patterns stored (see Fig. 14 in [10]). Recall dropped with a cal formalism of the model has been described elsewhere [10]. larger number of patterns stored due to interference from pre- Schematic representations of the model cells are depicted in viously stored memories. Proximal dendritic inhibition was Supplementary Figures 1–2. The dimensions of the somatic, held constant as the number of memory patterns stored was axonic and dendritic compartments of the model cells are pre- increased. sented in Supplementary Table 1. The parameters of all passive In this article, the Cutsuridis et al. [10] model is extended to and active ionic conductances used in the model are listed in uncover the biophysical factors that will improve its memory Supplementary Tables 2–4. The synaptic waveform parameters capacity and recall performance. In contrast to previous are given in Supplementary Table 5, and synaptic conductances models [7–10] where the strength of the bistratified cell are listed in Supplementary Table 6. Experimental support of the (BSC) inhibition to pyramidal cell (PC) dendrites was held choices of the various parameters is found in supplementary constant, the present model examined how selective modula- online material (SOM). tion of feedforward and feedback excitatory and inhibitory pathways targeting BSC and/or PCs may influence the BSC thresholding capacity to remove spurious activity and improve Pyramidal Cells the mean recall quality of PCs as a larger number of memories is stored. Each PC had 15 compartments (see Supplementary Figure S1), each containing a calcium pump and buffering mechanism, calcium-activated slow AHP and medium AHP K+ currents, an HVA L-type Ca2+ current, an HVA R-type Materials and Methods Ca2+ current, an LVAT-type Ca2+ current, an h current, a fast sodium and a delayed rectifier K+ current, a slowly Figure 1 in the main text illustrates the simulated microcircuit inactivating M-type K+ current and a fast inactivating A- model of the CA1 network. The model consists of 100 PCs, type K+ current [27, 28]. one axo-axonic cell (AAC), two basket cells (BCs), 1 BSC Each PC’s soma rested in the stratum pyramidale (SP), and one oriens lacunosum-moleculare (OLM) cell. All simu- while its dendrites extended across the strata from stratum lations were performed using NEURON [17] running on a PC oriens (SO) to stratum radiatum (SR) and stratum with four CPUs under Windows 8. lacunosum-moleculare (SLM). Each PC received somatic Simplified morphologies including the soma, apical and basal synaptic inhibition from the BCs, mid-dendritic excitation dendrites and a portion of the axon were used for each cell type. from Schaffer collaterals (CA3-PCs), proximal excitation The biophysical properties of each cell were adapted from cell from around 1% of other CA1 PCs in the network (recurrent collaterals) [1, 3], axonic inhibition from the AACs, spatially- distributed (six contacts) proximal dendritic synaptic inhibi- tion from BSCs and distal synaptic inhibition on each SLM dendritic branch from the OLM cell. Axo-Axonic Cell Each AAC had 17 compartments (see Supplementary Figure S2), which included a leak conductance, a sodium current, a fast delayed rectifier K+ current, an A-type K+ cur- rent, L- and N-type Ca2+ currents, a Ca2+-dependent K+ cur- rent and a Ca2+- and voltage-dependent K+ current [29]. Each AAC soma rested primarily in the SP, while its dendrites ex- tended across the strata from SO to SR and SLM. AACs Fig. 1 Hippocampal CA1 microcircuit showing major cell types and their connectivity. PC pyramidal cell, AAC axo-axonic cell, BC basket cell, received excitatory inputs from the CA3 Schaffer collateral BSC bistratified cell, EC entorhinal cortex input, CA3 CA3 Schaffer to their SR dendrites. In addition, the axo-axonic cells re- collateral input, MS1 medial septum cell population 1 (firing at theta ceived inputs from active CA1 pyramidal cells in their SR trough), MS2 medial septum cell population 2 (firing at theta peak). medium and thick dendritic compartments as well as inhibito- Black arrows: active excitatory input; light gray arrows: inactive excit- atory input; black filled circles: active inhibitory input; light gray filled ry input from the septum in their SO thick dendritic compart- circles: inactive inhibitory input ments [3, 13]. Cogn Comput (2019) 11:644–655 646 Basket Cell frequency of 8 Hz for a half-theta cycle (70 ms) followed by a half-theta cycle of silence. Due to 8% noise in the inter-spike Each BC had 17 compartments (see Supplementary intervals, the 10 spike trains in each septal population were Figure S2), containing a leak conductance, a sodium current, asynchronous. MS2 input provided GABA-A inhibition to a fast delayed rectifier K+ current, an A-type K+ current, L- BSCs and OLMs during the storage cycle (not simulated here; and N-type Ca2+ currents, a Ca2+-dependent K+ current and a see Fig. 3), whereas MS1 input provided GABA-A inhibition Ca2+- and voltage-dependent K+ current [29]. All BCs’ somas to AACs and BCs during the retrieval cycle (see Fig. 3). rested in SP, whereas their dendrites extended from SO to SLM. All BCs received excitatory connections from the CA3 Schaffer collaterals to their medium SR dendrites and Synaptic Properties from active pyramidal cells to their medium and thick SR dendritic compartments and inhibitory connections from In the model, AMPA, NMDA, GABA-A and GABA-B syn- neighbouring BCs and BSCs in their soma [12] and from the apses were considered. GABA-A were present in all strata, medial septum in their SO thick dendritic compartments. whereas GABA-B were present in medium and distal SR and SLM dendrites.
Details
-
File Typepdf
-
Upload Time-
-
Content LanguagesEnglish
-
Upload UserAnonymous/Not logged-in
-
File Pages12 Page
-
File Size-