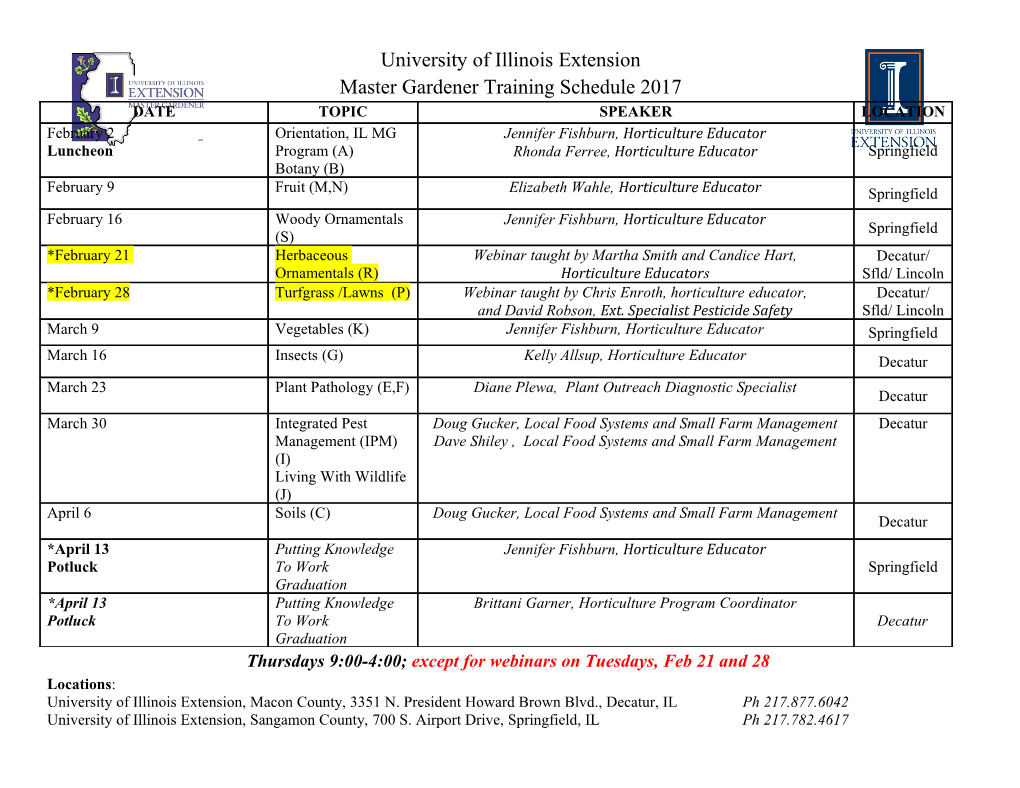
University of Central Florida STARS Electronic Theses and Dissertations, 2004-2019 2017 Thermal and Waveguide Optimization of Broad Area Quantum Cascade Laser Performance Matthew Suttinger University of Central Florida Part of the Electromagnetics and Photonics Commons, and the Optics Commons Find similar works at: https://stars.library.ucf.edu/etd University of Central Florida Libraries http://library.ucf.edu This Masters Thesis (Open Access) is brought to you for free and open access by STARS. It has been accepted for inclusion in Electronic Theses and Dissertations, 2004-2019 by an authorized administrator of STARS. For more information, please contact [email protected]. STARS Citation Suttinger, Matthew, "Thermal and Waveguide Optimization of Broad Area Quantum Cascade Laser Performance" (2017). Electronic Theses and Dissertations, 2004-2019. 6088. https://stars.library.ucf.edu/etd/6088 THERMAL AND WAVEGUIDE OPTIMIZATION OF BROAD AREA QUANTUM CASCADE LASER PERFORMANCE by MATTHEW MICHAEL SUTTINGER B.S. University of Central Florida, 2013 A thesis submitted in partial fulfillment of the requirements for the degree of Master of Science in the The College of Optics & Photonics at the University of Central Florida Orlando, Florida Fall Term 2017 Major Professor: Arkadiy Lyakh ©2017 Matthew Michael Suttinger ii ABSTRACT Quantum Cascade Lasers are a novel source of coherent infrared light, unique in their tunability over the mid-infrared and terahertz range of frequencies. Advances in bandgap engineering and semiconductor processing techniques in recent years have led to the development of highly efficient quantum cascade lasers capable of room temperature operation. Recent work has demonstrated power scaling with broad area quantum cascade lasers by increasing active region width beyond the standard ~10 μm. Taking into account thermal effects caused by driving a device with electrical power, an experimentally fitted model is developed to predict the optical power output in both pulsed and continuous operation with varying device geometry and minor changes to quantum cascade laser active region design. The effects of the characteristic temperatures of threshold current density and slope efficiency, active region geometry, and doping, on output power are studied in the model. The model is then used to refine the active region design for increased power out in continuous operation for a broad area design. Upon testing the new design, new thermal effects on rollover current density are observed. The model is then refined to reflect the new findings and more accurately predict output power characteristics. iii This work is dedicated to my wife, Kehan Yu, who is likely the only person outside of my committee who would ever bother to read it in its entirety. iv ACKNOWLEDGMENTS This work was accomplished with the help of Arkadiy Lyakh, the principal investigator of the projects that this work covers, the designer of the quantum well superlattices that are used, and a provider of guidance and advise throughout the process of this work. Ankesh Todi and his work in his master’s thesis provided initial data on power scaling with quantum cascade laser active region ridge width. Processing efforts by Pedro Figueiredo, Rowel Go, and Jason Leshin provided the devices that this thesis discusses. Justin Cook provided formatting help and guidance with the writing of this thesis. v TABLE OF CONTENTS LIST OF FIGURES ......................................................................................................... viii LIST OF ACRONYMS/ABBREVIATIONS .................................................................. xiv CHAPTER 1: INTRODUCTION ....................................................................................... 1 CHAPTER 2: OVERVIEW OF QUANTUM CASCADE LASER THEORY .................. 3 Section 1: Quantum Confinement ................................................................................... 3 Section 2: Lasers ............................................................................................................. 6 Section 3: Superlattice Design and Characteristics ........................................................ 8 Section 4: Waveguides .................................................................................................. 10 Section 5: Pulsed Characteristics .................................................................................. 14 Section 6: 1/L Measurements........................................................................................ 17 Section 7: Thermal Effects............................................................................................ 18 Section 8: Continuous Wave Operation ........................................................................ 19 CHAPTER 3: INITIAL DESIGN MEASUREMENTS ................................................... 20 Section 1: Quantum Cascade Laser Growth and Processing ........................................ 20 Section 2: Device Measurements .................................................................................. 21 CHAPTER 4: THE SEMIEMPIRICAL MODEL ............................................................ 25 Section 1: Waveguide Simulations ............................................................................... 25 Section 2: Pulsed Power................................................................................................ 26 Section 3: Continuous Wave Threshold ....................................................................... 27 Section 4: Continuous Wave Power ............................................................................. 28 vi Section 5: Replication of Experimental Data................................................................ 29 Section 6: Design Optimization for the Base Active Region Stage Design ................. 32 Section 7: Characteristic Temperature Analysis ........................................................... 33 Section 8: Separate Confinement Heterostructure ........................................................ 34 Section 9: Short Injector Design ................................................................................... 35 CHAPTER 5: SECOND DESIGN EXPERIMENTAL RESULTS AND THE REFINING OF THE MODEL ......................................................................................................................... 38 Section 1: Design Changes ........................................................................................... 38 Section 2: Characteristic Temperature .......................................................................... 39 Section 3: Rollover Current .......................................................................................... 40 Section 4: Model Refinements ...................................................................................... 41 CHAPTER 6: CONCLUSIONS ....................................................................................... 45 LIST OF REFERENCES .................................................................................................. 47 vii LIST OF FIGURES Figure 1: A single, finite, 1D quantum well with two confined states. The vertical axis represents energy level (potential energy in black, eigenenergy for the state as a dashed line.) The horizontal axis is position along the growth direction, z. Wavefunctions for each state are plotted as normalized curves anchored with 0 probability density at their energy levels (dashed lines.) The first (red) and second (green) order modes are the two confined states allowed by this quantum well. ................................................................................................................................................. 4 Figure 2: A double quantum well potential. First and second order states for the coupled wells displayed as wavefunctions anchored at state energy (shown as dashed lines) for 0 probability density. The lower modes in red and green show the coupled first order modes for the two interacting wells, with energy levels separated by a small amount. The upper modes in red and blue are coupled second order modes, slightly more separated in energy. ..................................... 5 Figure 3: Illustration of the simplified two level model for a quantum cascade. The potential, shown in black, is a series of quantum wells, coupled by proximity. The slope is introduced as a voltage bias contributing to potential energy for the electron experiencing the potential. Representations of wavefunction locations (horizontal position) and energy levels (vertical position) are shown as the red and blue upper and lower energy levels. Lasing is modelled as stimulated emission from an upper to lower laser level within a well. Electrons tunnel through barriers from lower levels in one well to the upper level in the next well with lower potential energy due to the voltage bias..................................................................................................................... 7 Figure 4: The active region design used for the first iteration of devices tested in this work. The potential energy structure making up the quantum well superlattice is shown as the thin black viii lined background pattern. The superlattice is a series of Aluminum Indium Arsenide (barriers) and Indium Gallium Arsenide (wells) layers biased by a voltage to introduce the slope in the potential porfile. Curves represent wavefunctions generated by this potential. Wavefunctions are anchored at their state energy,
Details
-
File Typepdf
-
Upload Time-
-
Content LanguagesEnglish
-
Upload UserAnonymous/Not logged-in
-
File Pages62 Page
-
File Size-