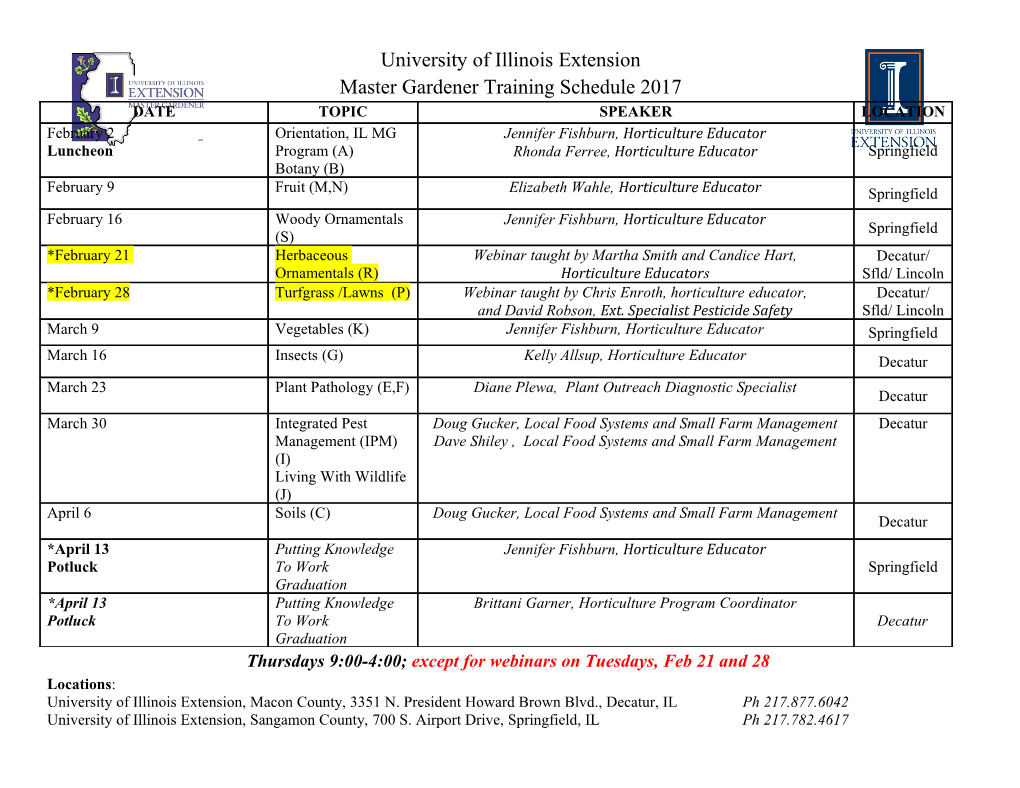
Neural interfaces for Implantable Neuroprosthetics & Neuromodulation Daryl R. Kipke, Ph.D. ([email protected]) Professor, Biomedical Engineering University of Michigan, Ann Arbor, Michigan USA Neural Engineering Laboratory nelab.engin.umich.edu Center for Neural Communication Technology (CNCT) cnct.engin.umich.edu NIH/NIBIB Biotechnology Resource Center; P41-EB002030 May 2009 D. Kipke, Center for Neural Communication Technology (http://cnct.engin.umich.edu) Disclosure: D. Kipke has a financial and leadership interest in NeuroNexus Technologies, Inc. Microscale Neural Interfaces 1 mm 2 mm ~60,000 neurons 1.4x109 synapses Breakthrough neurotechnology (clinical, scientific) – Permanent, high-fidelity, high-bandwidth neural interface – Multi-modal interfaces Electrical AND chemical sensing/actuation – Integrated microsystems for “smart” neural interface Interplay between neuroscience, technology & clinical applications Science drivers: Increased Technology drivers: Increased understanding of normal, diseased, and neural interface selectivity, injury mechanisms in brain sensitivity, fidelity, bandwidth Science pull Technology push Advanced, Microfabricated Electrode Arrays • Conventional microelectrodes: glass or metal, single tip site • Microfabricated electrode – 64 recording and/or stimulation on same sized or smaller carrier than a conventional single channel microelectrode • Attributes – Multiple channels – Batch fabricated – High reproducibility – Any 2-D shape – Precise dimensions – Biocompatible materials Manufacturing of Planar Microfabricated Electrode Arrays Sites Leads (Neural interface) (Signal transfer) Iridium Polysilicon Gold Gold Platinum Platinum TiN Substrate Dielectrics (Structural support) (Lead insulation, Silicon structural support) Ceramic SiO2 Polyimide Si3N4 Parylene SiC PDMS Polyimide Parylene PDMS PTFE Survey of Microelectrode Array Technology 7 Technology Base Technology Site coatings 3D array assembly Wise, et al. IEEE Trans. Biomed. Eng., 1970. Buried fluidic channels Silicon ribbon cables Site formation process Boron doped substrate NIH contract to Michigan 1st microfabricated array 1960 1970 1980 1990 2000 2010 Technology Base Technology Site coatings Najafi, et al., IEEE Trans. Elec. Dev., 1985. 3D array assembly Buried fluidic channels Silicon ribbon cables Site formation process Boron doped substrate NIH contract to Michigan 1st microfabricated array 1960 1970 1980 1990 2000 2010 Technology Base Technology Application Space Site coatings 3D array assembly Buried fluidic channels Silicon ribbon cables Spinal cord stim Chronic recording Auditory mapping Cardiac tissue Site formation process Brain response to Si Insect antennal lobe Boron doped substrate CNCT I began NIH contract to Michigan 1st CSD application paper 1st microfabricated array 1960 1970 1980 1990 2000 2010 1st probe shipped to external user, 1st detailed analysis of recording capability Implantable Michigan Probes Basic probe assembly for chronic studies in animals Vetter, Kipke, et al. (2004) IEEE Trans Biomed Eng Kipke et al. (2003). IEEE Trans Neural Systems and Rehab. Engin. Multi-site Neural Recordings: Spikes & Local Field Potentials Buzsaki 2003 A. Schwartz A CNCT II began CNCT I ended, NeuroNexus Technology Base Technology launched Application Space Site coatings 3D array assembly Buried fluidic channels Silicon ribbon cables Spinal cord stim Chronic recording Auditory mapping Site formation process Cardiac tissue Brain response to Si Insect antennal development clinical device lobe Boron doped substrate CNCT I began Long-term neural interface and NIH contract to Michigan 1st CSD application paper 1st microfabricated array 1960 1970 1980 1990 2000 2010 1st probe shipped to external user, 1st detailed analysis of recording capability Center for Neural Communication Technology Biotechnology Resource Center supported by the NIH NIBIB Mission: Develop microscale neural probe technologies to enable chronic, high-fidelity neural interfaces to the CNS Objectives: ! Develop implantable neural probes for multichannel chronic electrical and chemical interfaces ! Characterize and control long-term device biocompatibility ! Provide service and training to facilitate technology development with collaborators and users ! Disseminate research and technology outcomes to broad neuroscience, neurology, and bioengineering communities Conductive Polymer (PEDOT) Electrode Site Coatings for Improved Recording Characteristics Ludwig et al, J Neural Engin., 2006 Abidian et al, IEEE Conf. on Neural Engineering, 2007 15 Ultra-small edge electrodes for neural recording Seymour & Kipke, Biomaterials, 2007 Parylene-based Edge Electrodes 80 !m2 footprint Sub-cellular parylene edge 5 !m thick Edge electrode has site area ~100 um2 Seymour & Kipke, In preparation (2008) Biomaterials 2007 Parylene-based Edge Electrodes Summary of Recordings Example Recordings Seymour & Kipke, In preparation (2008) Interplay between neuroscience, technology & clinical applications Science drivers: Increased Technology drivers: Increased understanding of normal, diseased, and neural interface selectivity, injury mechanisms in brain sensitivity, fidelity, bandwidth Clinical drivers: Meeting specific and significant clinical needs Standard and customized Global marketing and sales to neural interface products neuroscience researchers Clinical translation: Innovative Deep-Brain Mapping Array to improve surgical targeting Partnership with FHC, Inc. Clinical translation: Innovative implantable DBS system to improve DBS therapy Press Release (Nov 2008) “NeuroNexus Technologies and Philips partner to research next-generation deep brain stimulation devices for the treatment of central nervous system disorders” • Multi-directional stimulation • Miniaturized, cranial chamber • Wireless and rechargeable • MR-safe • Segmented thin-film electrodes • (outpatient) Neural recording 23.
Details
-
File Typepdf
-
Upload Time-
-
Content LanguagesEnglish
-
Upload UserAnonymous/Not logged-in
-
File Pages23 Page
-
File Size-