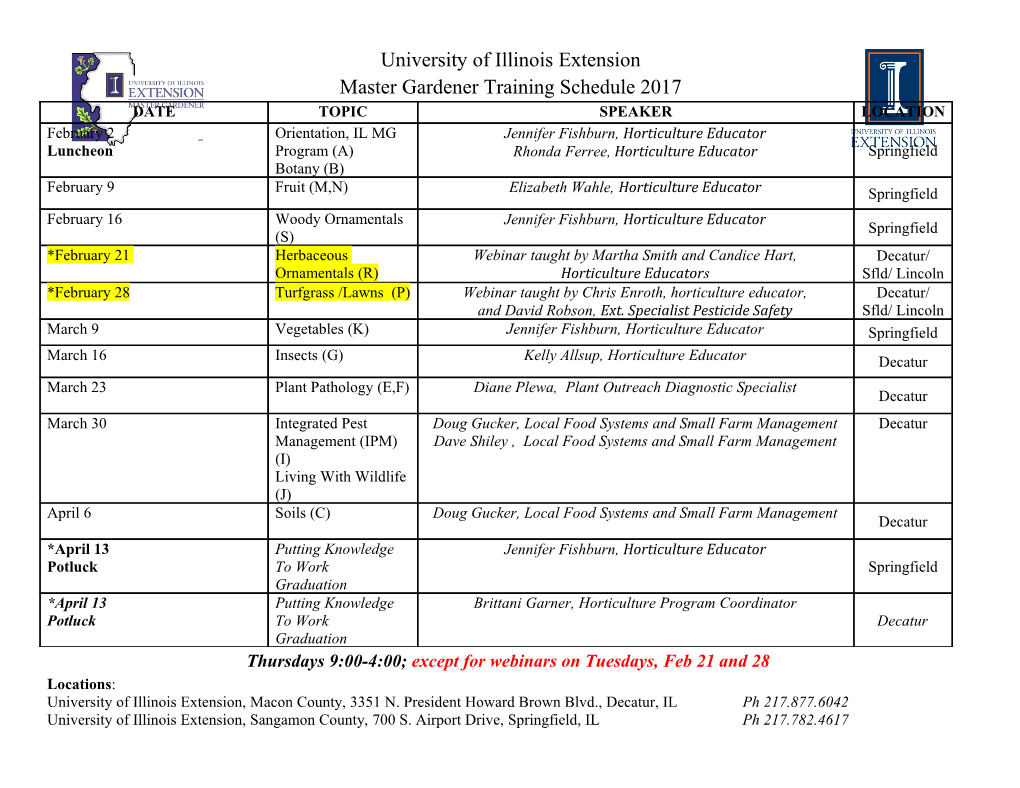
Predictive Theory and Modelling of Heterogeneous Interfaces By Tuan Anh Pham B.S. (Hanoi National University of Education) 2006 DISSERTATION Submitted in partial satisfaction of the requirements for the degree of DOCTOR OF PHILOSOPHY in CHEMISTRY in the OFFICE OF GRADUATE STUDIES of the UNIVERSITY OF CALIFORNIA DAVIS Approved by: Professor Giulia Galli (Chair) Professor C. William McCurdy Professor Gergely Zimanyi Committee in Charge 2014 i c Tuan Anh Pham, 2014. All rights reserved. For my mother, my dad and for my wife, for all their support and encouragement along the way. And for my awaited son for the great motivation. ii Contents Abstract viii Acknowledgements and Thanks x List of Figures xv List of Tables xvii Introduction 1 1 Theoretical background 6 1.1 Schr¨odingerequation and Born-Oppenheimer approximation . .6 1.2 Density functional theory . .8 1.3 First-principles molecular dynamics . 11 1.4 Many-body perturbation theory . 13 1.4.1 Green's function . 14 1.4.2 Dyson's equation and the GW approximation . 15 2 Improving the calculation of photoelectron spectra within many-body perturbation theory 18 2.1 Introduction . 18 2.2 Implementations of the GW approach . 20 iii 2.3 GW approach using the spectral decomposition of the dielectric matrix . 23 2.3.1 Iterative calculation of dielectric eigenpotentials . 23 2.3.2 The self-energy operator . 25 2.3.3 Lanczos algorithm . 27 2.3.4 Computational cost . 29 2.4 Benchmark for the benzene molecule . 30 2.4.1 Convergence properties . 30 2.4.2 Basis functions . 34 2.4.3 Analytic continuation . 36 2.5 Comparison with other GW implementations . 37 2.5.1 Comparison with plane-wave basis set implementations . 37 2.5.2 Comparison with localized basis set implementations . 40 2.6 Ionization potentials of the test set G2/97 . 42 2.7 Band gaps of semiconductors . 45 2.7.1 Quasiparticle energies of Si, AlAs and SiC . 45 2.7.2 Band gaps of amorphous Si3N4 .................... 47 2.8 Quasiparticle energies of diamondoids . 50 2.9 Summary . 53 3 Structural and dynamical properties of oxides surfaces and interfaces 54 3.1 Ice Ih (0001) surface . 54 3.1.1 Introduction . 54 3.1.2 Methods . 56 3.1.3 Results and Discussion . 59 3.1.4 Summary . 69 3.2 Al2O3/water interface . 69 3.2.1 Introduction . 69 iv 3.2.2 Methods . 72 3.2.3 Interface model . 74 3.2.4 Results and discussion . 75 3.2.5 Summary . 88 4 Electronic and dielectric properties of nitride surfaces and interfaces 90 4.1 Dielectric properties of Si3N4 thin films . 90 4.1.1 Introduction . 90 4.1.2 Methods . 91 4.1.3 Results and Discussion . 93 4.1.4 Summary . 98 4.2 Band offsets and dieletric properties of the Si3N4/Si(100) interface . 98 4.2.1 Introduction . 98 4.2.2 Methods . 100 4.2.3 Results and Discussion . 101 4.2.4 Summary . 106 5 Electronic properties of simple aqueous solutions 108 5.1 Electronic structure of liquid water . 108 5.1.1 Introduction . 108 5.1.2 Methods . 110 5.1.3 Results and Discussion . 112 5.1.4 Summary . 119 5.2 Electronic structure the solvated chloride anion . 120 5.2.1 Introduction . 120 5.2.2 Methods . 121 5.2.3 Results and Discussion . 122 v 5.2.4 Summary . 126 6 Electronic properties of semiconductors interfaced with liquid water 127 6.1 Introduction . 127 6.2 Calculations of Semiconductor Band Edges . 131 6.3 Application to Si(111) surfaces . 134 6.3.1 Computational Details . 134 6.3.2 Results and Discussions . 136 6.4 Summary . 146 Conclusions 148 List of publications 152 References 155 vi Tuan Anh Pham September 2014 Chemistry Predictive Theory and Modelling of Heterogeneous Interfaces Abstract While much progress has been made in the microscopic characterization of bulk materials, understanding the behavior, at the atomic scale, of complex materials interfaces remains a challenging problem in condensed matter physics and materials science. Development of predictive theory and modeling for interfacial systems is not only important to aid experi- mental interpretations but is also crucial to accelerate materials design efforts for a broad range of technological applications from the semiconductor industry to the development of new sources of energy. This dissertation presents a computational framework that combines first-principles molecular dynamics simulations for the determination of interfacial atomic structures, and advanced electronic structure methods for the description of electronic properties to understand, predict and design materials interfaces. In particular, the research presented in this thesis was carried out in two parallel directions: • Predictions of the interfacial atomic structure of complex materials interfaces using first-principles molecular dynamics, and validation of the predictions by relating structural models to experiments, e.g., data from X-ray, infrared spectra and sum- frequency generation spectroscopy experiments. In particular, I investigated structural and dynamical properties of the ice Ih sur- face and of the Al2O3/water interface. While the study of the ice surface is the first step towards the understanding of complex semiconductor/water interfaces, the Al2O3/water interface represents a suitable prototype interface for extensive comparisons between theory and experiments. vii • Development of advanced first-principles techniques to study electronic states at interfaces and application of these techniques to gain insights on the relationship between between local interface structure and electronic properties. I devised a new technique to study excited states and photoemission spectra based on many-body perturbation theory, within the so-called GW approximation, which improves both the computational efficiency and accuracy of existing methodologies, and that can be employed to study realistic systems. In addition, in the thesis I employed the new GW technique to investigate a variety of systems including molecules, nanostructures, semiconducting interfaces, liquid water and simple aqueous solutions. These studies are crucial to build a fundamental understanding of the electronic structure of semiconductor/liquid water interfaces and of, e.g., water with dissolved ions under different pH conditions, interfaced with a photoelectrode. Information provided by these two parallel research directions helped establish a structure- electronic properties-chemical reactivity paradigm, that is general and applicable to a large class of materials. An example presented in the thesis is that of functionalized Si surfaces interfaced with liquid water, in which I studied the effect of surface functionaliza- tion on the alignment between Si band edges and water redox potentials, and I suggested a possible approach to engineer and design semiconductor surfaces for photoelectrochemical water splitting. viii Acknowledgments and Thanks I would like to express my deepest gratitude to a number of people without whom this thesis would not be completed. First and foremost, I would like to thank the support and direction I received from my advisor, Prof. Giulia Galli. During the last five years of my PhD, Giulia has pro- vided me with an excellent research environment, offering me the opportunity to work with outstanding researchers, and more importantly, the opportunity to explore ideas independently. Giulia's incredible passion for scientific research, her vision and optimism have had an enormous impact on my study as well as on my future career. I would like to thank Dr. Eric Schwegler, my mentor at the Lawrence Livermore National Laboratory, for his priceless guidance, encouragement and constant support. Thanks to Eric, I have gradually developed my professonalism as well as improved my self-development. I would like to thank my collaborators: Prof. Francois Gygi, Prof. Tianshu Li, Dr. Huy Viet Nguyen, Dr. Dario Rocca, Dr. Cui Zhang, Dr. Deyu Lu, Dr. Patrick Huang, Dr. Donghwa Lee, Dr. Daniel Opalka, Prof. Michiel Sprik and Dr. Sadasivan Shankar for the fruitful work and delightful collaboration we had together. Without their support, this thesis would not be possible. I would like to thank all of the other Angstrom group members at the University of California Davis, and Quantum Simulations group members at the Lawrence Livermore ix National Laboratory for their tremendous support and encouragement. I I have learned so much from each of them, and I appreciate the pleasant times we spent together. Last but not least, my wife Linh Trinh holds all my gratitude for her patience, support and for her incredible faith in my talent. Her love has enriched everyday in my life with happiness and passion. This thesis is dedicated to her, my awaited son and my beloved parents. x List of Figures 2.4.1 Imaginary and Real part of the correlation self-energy computed for the HOMO level of the benzene molecule as a function of the imaginary frequency. 31 2.4.2 Differences between the calculated vertical ionization potentials of the ben- zene molecule and experimental results as a function of the number of eigenpotentials included in the definition of the dielectric matrix. 33 2.4.3 Imaginary and Real part of the correlation self-energy for the HOMO level of the benzene molecule, computed for different threshold used in the iter- ative diagonalization procedure. 35 2.4.4 Vertical ionization potential and electron affinity of the benzene molecule, computed for different number of pole included in the multipole model function. 37 2.6.1 Deviation of computed ionization potentials of a 80 molecule set, obtained within different approaches, with respect to experimental data. 44 2.8.1 Computed and measured ionization potentials of diamondoids and dia- mond clusters as a function of the number of constituent carbon atoms. 51 3.1.1 Models of the ice Ih (0001) surface: a proton ordered surface where the Fletcher phase is employed, and proton disordered surfaces. 57 3.1.2 Calculated infrared spectra of bulk regions of the two ice Ih slab models with proton disordered and ordered surfaces.
Details
-
File Typepdf
-
Upload Time-
-
Content LanguagesEnglish
-
Upload UserAnonymous/Not logged-in
-
File Pages194 Page
-
File Size-