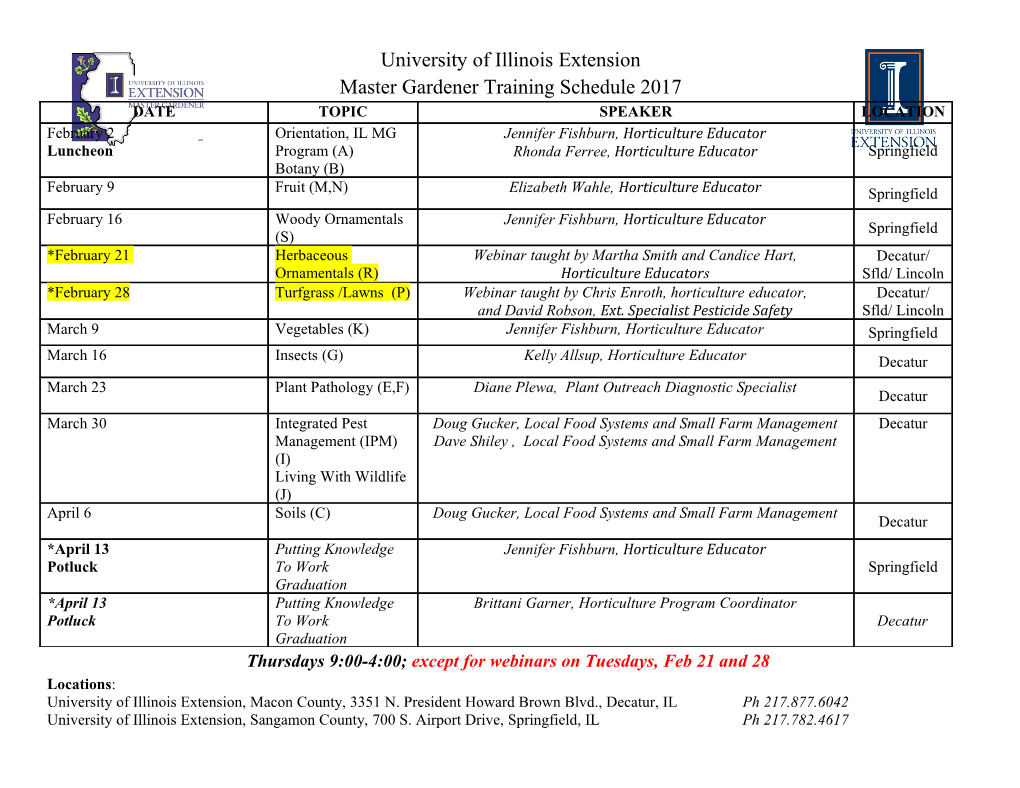
The Future Circular Collider (FCC) project and its cryogenic challenges Laurent Tavian, CERN ECD 2017, Karlsruhe, 13 September 2017 Content • Introduction: Scope of the FCC study • FCC-hh tunnel cryogenics and user heat loads • FCC-hh cryogenics layout and architecture • FCC-hh cool-down and nominal operation • FCC-hh electrical consumption and helium inventory • Conclusion Scope of FCC Study International FCC collaboration (CERN as host lab) to study: • pp-collider (FCC-hh) main emphasis, defining infrastructure requirements ~16 T ⇒ 100 TeV pp in 100 km • ~100 km tunnel infrastructure in Geneva area, site specific • e+e- collider (FCC-ee), as potential first step • p-e (FCC-he) option, integration one IP, e from ERL • HE-LHC with FCC-hh technology • CDR for end 2018 Luminosity vs energy of colliders Conventional He I He II 1.E+36 x 7 FCC 1.E+35 HL-LHC ] x 30 1 - LHC 2017 .s 2 1.E+34 - LHC (design) 1.E+33 ISR 1.E+32 LEP2 HERA TeVatron Luminosity [cm LEP1 1.E+31 SppS 1.E+30 0.01 0.1 1 10 100 Centr-of-mass energy [TeV] CERN Collider plan 1980 1985 1990 1995 2000 2005 2010 2015 2020 2025 2030 2035 2040 Construction Physics Upgr LEP Design Proto Construction Physics LHC Design Construction Physics HL-LHC Future Collider Design Proto Construction Physics ~25 years FCC-hh baseline parameters Parameter LHC HL-LHC FCC-hh c.m. energy [TeV] 14 100 Nb3Sn superconducting dipole magnet field [T] 8.33 16 magnets cooled at 1.9 K circumference [km] 26.7 100 luminosity [1034 cm-2.s-1] 1 5 5 29 bunch spacing [ns] 25 25 event / bunch crossing 27 135 170 ~50 mm bunch population [1011] 1.15 2.2 1 norm. transverse emittance [µm] 3.75 2.5 2.2 IP beta-function [m] 0.55 0.15 1.1 IP beam size [µm] 16.7 7.1 6.8 synchrotron rad. [W/m/aperture] 0.17 0.33 28 critical energy [keV] 0.044 4.3 5 MW dissipated in cryogenic environment total syn. rad. power [MW] 0.0072 0.0146 4.8 beam screens are mandatory longitudinal damping time [h] 12.9 0.54 Cooling temperature 40-60 K Magnet cooling cost including 10 years of operation 1 He II cooling Min Max [a.u.] Magnet cooling at 1.9 K vs 4.5 K: About a factor 2 on the magnet 0.5 cooling cost largely compensated by the saving on superconducting material Tmagnet = 1.9 K He I cooling FCC magnet cooling cost cost cooling magnet FCC 0 1.5 2 2.5 3 3.5 4 4.5 5 Magnet temperature [K] Beam screen – cold mass thermodynamics Cold bore (Tcm) Ta: Ambient temperature Qbs Cooling channel (Tbs) Support Qcm Qsr Energy balance: beam Qbs = Qsr - Qcm Beam screen (Tbs) - Exergy load ∆E = measure of (ideal) refrigeration duty : ∆E = ∆Ecm + ∆Ebs ∆E = Qcm . (Ta/Tcm – 1) + Qbs . (Ta/Tbs – 1) - Real electrical power to refrigerator: Pref= ∆E/η(T) with η(T) = efficiency w.r. to Carnot = COPCarnot/COPReal Pref = Qcm . (Ta/Tcm – 1)/η(Tcm) + Qbs . (Ta/Tbs – 1)/η(Tbs) BS – CM thermodynamics Numerical application 3000 Total electrical power to refrigerator Pref. considering: 2500 - a beam screen similar to that of the LHC - refrigerator efficiencies identical to those 2000 of the LHC. 1500 Optimum for Tbs= ~ 70 K 1000 500 Temperature range 40-60 K retained per beam] [W/m to refrigerator power Total 0 0 50 100 150 200 Beam-screen temperature, Tbs [K] Forbidden by vacuum and/or by surface impedance FCC-hh: tunnel cryogenics Main distribution based on INVAR® technology Contribution of WUST Specific heat loads at different temperature levels Temperature level 40-60 K 1.9 K 4 K VLP CM supporting system 2 0.13 Radiative insulation 0.13 Thermal shield 3.1 Static heat in-leaks Feedthrough & vacuum barrier 0.2 0.1 [W/m] Beam screen 0.12 Distribution 4 0.1 0.24 Total static 9.3 0.58 0.24 Synchrotron radiation 57 0.08 Image current 3.4 Dynamic heat loads Resistive heating in splices 0.3 [W/m] Beam-gas scattering 0.45 Total dynamic 60 0.83 Total [W/m] 70 1.4 0.24 Dynamic range [-] 8 2.5 1 FCC-hh cryogenic layout and architecture 40-60 K 1.9 K 4 K VLP 40-300 K [kW] [kW] [kW] [g/s] 580 12* 2 85 Without operational margins as the working conditions has to be considered as ultimate *: Outside State-of-the-Art State-of-the-art of cold compressors (single train) 12 FCC-hh 10 8 Increase by a factor 5 on the cooling power, i.e with respect to the present technology: 6 Impeller diameter from 350 mm to 700 mm (factor 2) 4 LHC Shaft power from 10 kW to 30 kW (factor 3) Total cooling Total cooling power [kW] 2 0 1.6 1.7 1.8 1.9 2 2.1 Saturated temperature [K] FCC-hh cryoplant architecture C C C C C C Quench buffer • Beam screen (40-60 K) Ne-He T • Thermal shield (40-60 K) 300-40 K • Current leads (40-300 K) C • Precooling of 1.9 K cryoplant T cryoplant Surface LHe storage Shaft T Cavern He 1.8 K • SC magnet cold mass T cryoplant T Tunnel E F C Qb B D Qts Qcl Qcm Contributions of TU Dresden and CEA/SBT CM Qbs Qts Ne-He cycle: 750-1000 kW between 40 and 60 K C Hermetically sealed centrifugal compressors: - No dry gas seals, no lube-oil system and no gearbox Turbo- - Use of high speed induction motor (up to 200 Hz) T Brayton and active magnetic bearings. The motor is cooled by cycle process gas and directly coupled to the barrel type Courtesy of MAN Diesel & Turbo compressor. C T Difficult to get high compression ratio and high compression efficiency with pure helium (light mono-atomic gas): Compression of a mixture of helium and neon (~75-25 %) (OK with neon as refrigeration T > 40 K) The warm compression efficiency is improved Expected global efficiency with respect to Carnot 42 % Cryogenics (CERN), L. Tavian, 1st Sept’17 Cooldown of FCC-hh C C C C C C Quench He cycle buffer Cool-down capacity produced Ne-He cycle by the Ne-He cycle: No need of LN2 cool-down T unit with its huge LN2 storage and logistics C T Cold mass: 2.8 t/m i.e. Surface 23 kt per sector LHe storage 230 kt for FCC Shaft T Cavern 15 days of cool-down time T from 300 to 40 K (10 days (on paper) with LN2). T 50 bar supply pressure Cost (only energy) indirect cool- Qb down of cold- Full FCC CD 2.3 MCHF Tunnel E F C B D masses (design (To be compared with the Qts pressure of 20 Qcl cost of a CD using LN2 Qcm bar) CM 45000 t, i.e. ~4 MCHF) Qbs Qts FCC-hh Nominal operation C C C C C C Quench C C C C C C Quench buffer He cycle buffer Ne-He cycle T T C C T BS cooling with cold circulator (+130 kW @ 40-60 K) T Surface Exegetic efficiency of BS cooling loop: 82 % Surface LHe storage LHe storage Shaft Shaft T BS cooling with warm circulator needed for cooldown T Cavern Cavern (+140 kW @ 40-60 K and +500 kW @ 300 K) T Less efficient (exergetic efficiency of 71 %) but good for T redundancy. T T Qb Tunnel E F C B D Tunnel E F C Qb B D 1.8 K refrigeration based on a mixed compression Qts cycle (cold centrifugal compressors in series with Qts Qcl Qcl Qcm warm volumetric compressors) Qcm CM CM Qbs Qts Qbs Qts FCC-hh Half-cell cooling loop Line B (4 K, 15 mbar) Line C (4.6 K, 3 bar) Line D (40 K, 1.3 bar) Line E (40 K, 50 bar) Line F (60 K, 44 bar) V2 Sub-cooling HX Distribution-line thermal shields V4 Bayonet HX V7 Feeder pipe V3 V6 V5 V1 Magnet thermal shields and support-post heat intercepts Beam 1 Beam 2 Q D D D D D D SC coils Beam screens Pressurized LHeII Half-cell length (~107 m) FCC-hh Superfluid helium cooling loop parameters Variable Unit LHC FCC Unit cooling length m 106.9 107.1 Sector cooling length m 2900 8400 Average heat load nominal capacity W/m 0.40 1.38 Bayonet HX inner diameter mm 53.4 83.1 Feeder pipe inner diameter mm 10.0 15.0 Thickness bayonet HX pipe wall mm 2.3 5.0 Joule-Thomson valve inlet temperature K 2.18 2.18 Free longitudinal cross-section area cm2 60 156 DT max Pressurized-saturated HeII mK 50 50 Cold mass operating pressure bar 1.3 1.3 Header B diameter mm 270 630 (500) Heat load on header B W/m 0.11 0.24 Pumping pressure at cryoplant interface mbar 15 15 Maximum cold-mass helium temperature K 1.9 1.9 (1.98) FCC-hh Beam-screen cooling loop parameters Main parameter Unit LHC FCC Transient modes Unit cooling length m 53.4 107.1 Sector cooling length m 2900 8400 - Working at constant nominal mass-flow to Average BS nominal capacity W/m 1.6 60 handle the severe transient during energy ramp-up Max. supply pressure bar 3 50 - Working at constant He Supply helium temperature K 5 40 inventory to avoid big mass discharge and refill (~6 t) Max.
Details
-
File Typepdf
-
Upload Time-
-
Content LanguagesEnglish
-
Upload UserAnonymous/Not logged-in
-
File Pages25 Page
-
File Size-