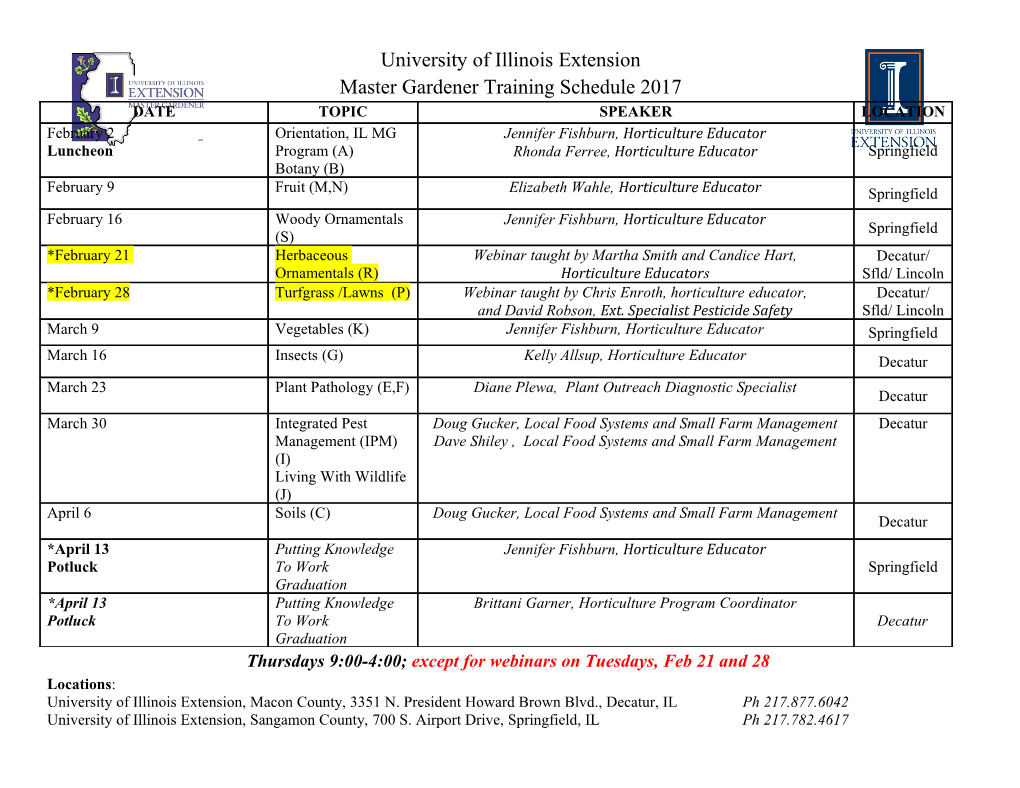
REVIEW & INTERPRETATION Molecular Markers and Selection for Complex Traits in Plants: Learning from the Last 20 Years Rex Bernardo* Dep. of Agronomy and Plant Genetics, Univ. of Minnesota, 411 Bor- ABSTRACT laug Hall, 1991 Upper Buford Cir., Saint Paul, MN 55108. Received 7 In the mid-1980s, the development of abundant Mar. 2008. *Corresponding author ([email protected]). molecular markers, appropriate statistical pro- Abbreviations: BLUP, Best linear unbiased prediction; QTL, quan- cedures, and user-friendly computer software titative trait loci; RFLP, restriction fragment length polymorphisms; that implemented these statistical procedures SNP, single nucleotide polymorphisms. permitted the detection of molecular markers associated with quantitative trait loci (QTL) for complex traits. Marker-assisted selection was n the last two decades we have witnessed the widespread then proposed as a means of exploiting mark- Iuse of molecular markers to study complex, quantitative traits ers linked to QTL to develop improved cultivars. in diff erent crop species. My thesis in this Perspectives article is But while thousands of marker-trait associations that while many QTL have been reported in the literature, these have been reported for many traits in different reported QTL have not been adequately exploited in breeding plant species, far fewer examples of success- programs. My objectives are to discuss practical lessons that I fully exploiting mapped QTL have been reported think we, as a scientifi c community of plant breeders and geneti- in the literature. Key lessons learned from apply- cists, have learned about marker-based selection for quantitative ing markers in plant breeding include the follow- traits in the last 20 years, and to present insights on how we might ing: (i) the purpose of detecting QTL should be best use molecular markers to improve complex traits in current clearly defi ned before embarking on QTL map- and future plant breeding programs. ping; (ii) procedures for marker-based selection depend on the number of QTL; (iii) estimates of QTL effects for complex traits are often incon- FROM PHENOTYPE TO (MARKER) GENOTYPE sistent; and (iv) gain per unit cost and time rather My view of plant breeding became more complicated one than gain per cycle should be considered. Future autumn day in 1986, when a Ph.D. student in molecular applications for complex traits will likely focus genetics walked into our basement offi ce at the University of on predictive methodologies for marker-based Illinois and began describing a promising new technology called selection before phenotyping and for marker- restriction fragment length polymorphisms, or RFLPs or “rifl ips” based selection without QTL mapping. These for short (Grodzicker et al., 1974). Back then I was a Ph.D. stu- applications will take advantage of cheaper dent in quantitative genetics and maize breeding with Profes- costs of genotyping than of phenotyping. sor John W. Dudley. Based on what my adviser had taught me, plant breeding for complex traits seemed fairly simple: a breeder (i) created genetic variation mainly by crossing good by good, (ii) Published in Crop Sci. 48:1649–1664 (2008). doi: 10.2135/cropsci2008.03.0131 © Crop Science Society of America 677 S. Segoe Rd., Madison, WI 53711 USA All rights reserved. No part of this periodical may be reproduced or transmitted in any form or by any means, electronic or mechanical, including photocopying, recording, or any information storage and retrieval system, without permission in writing from the publisher. Permission for printing and for reprinting the material contained herein has been obtained by the publisher. CROP SCIENCE, VOL. 48, SEPTEMBER–OCTOBER 2008 1649 selected the best progenies in the cross, and (iii) synthe- et al., 1993), JoinMap (Stam, 1993), QTL Cartographer (Bas- sized the best progenies into a new and improved cultivar ten et al., 1994), PLABQTL (Utz and Melchinger, 1996), (Dudley and Moll, 1969). The breeding decisions pertain- QGene (Nelson, 1997), and TASSEL (Buckler, 2007). ing to these tasks were made on the basis of phenotypic Private breeding companies in turn have developed their data. But this fellow graduate student showed us maize- own QTL analysis tools that are integrated with company breeding students a recent article that concluded that “the infrastructure for managing both marker and non-marker advent of RFLPs, by greatly increasing the total number of data (Eathington et al., 2007). polymorphic genetic markers available to the agricultural community, may signal the advent of a new and promising QTL, QTL EVERYWHERE era for the understanding and genetic improvement (emphasis Reports of mapped QTL are now pervasive in the plant mine) of quantitative economic traits through the use of breeding literature. To obtain a rough estimate of the num- marker-assisted breeding methodologies” (Beckmann and ber of QTL mapping studies that have been conducted Soller, 1986). to date, I searched the Web of Science database for titles Two parallel developments have allowed the aggres- that contained the terms (i) “QTL,” “QTLs,” “quantita- sive use of molecular markers for studying quantita- tive trait locus,” “quantitative trait loci,” or “markers” tive traits. First, new marker systems such as randomly + “associated” and (ii) the common names of 12 major amplifi ed polymorphic DNA, microsatellites or simple crop species [barley (Hordeum vulgare L.), bean (Phaseolus sequence repeats (SSR), amplifi ed fragment length poly- spp. and Vicia faba L.), corn or maize, cotton (Gossypium morphisms (AFLP; Vos et al., 1995), single nucleotide spp.), oat (Avena sativa L.), potato (Solanum tuberosum L.), polymorphisms (SNP), and diversity arrays technology rice (Oryza sativa L.), soybean [Glycine max L. (Merr.)], markers (DArT; Kilian et al., 2005) have increased the sorghum (Sorghum bicolor L.), sunfl ower (Helianthus spp.), number and decreased the cost of markers in diff erent tomato (Lycopersicon spp.), and wheat (Triticum aestivum crop species (Burrow and Blake, 1998; Bhattramakki and L.)]. This search indicated that more than 1200 QTL Rafalski, 2001). For example, the development of high- mapping studies have been reported for these 12 species. throughput technologies for SNP genotyping (Jenkins Previous reviews have indicated that QTL mapping stud- and Gibson, 2002; Syvänen, 2005) has led to, since 2000, ies have typically detected an average of 3 to 5 QTL for a 40-fold increase in the number of marker datapoints each trait (Kearsey and Farquhar, 1998; Bernardo, 2002, generated and a six-fold decrease in the cost per datapoint p. 309–311). If we assume that QTL mapping studies in a commercial maize (Zea mays L.) breeding program typically involve ~3 traits (as indicated by Kearsey and (Eathington et al., 2007). The U.S. small-grains breeding Farquhar, 1998), we can then surmise that at least 10,000 community is served by four USDA regional genotyping marker-trait associations in diff erent plant species have centers that have made marker genotyping routine (Chao been reported in the literature. et al., 2006), and diff erent molecular-marker service labs The reported QTL have typically accounted for a total in North America, Europe, and Australia provide con- of 40 to 60% of the phenotypic variance for the quantitative tractual genotyping services. trait, and the distribution of the estimated genetic eff ects Second, statistical methods for detecting QTL and of individual QTL has been consistent with a quantitative computer software for implementing these procedures trait being controlled by few QTL with large eff ects and have been developed. In this article I defi ne “QTL map- many QTL with small eff ects (Kearsey and Farquhar, 1998; ping” as the general class of linkage-based methods for Bernardo, 2002). Overall, the QTL mapping literature has fi nding QTL, typically in a cross between two inbreds shown that if a breeder can develop a mapping population (Dudley, 1993). Methods for QTL mapping range from of N = 100–150 progenies derived from an F2 or backcross the simplest method of single-marker analysis (Sax, 1923) population between two inbreds, obtain reasonably good to more sophisticated methods such as interval mapping phenotypic data for the traits of interest, and genotype (Lander and Botstein, 1989; Haley and Knott, 1992), joint the population with markers spaced about 10 to 15 cM mapping (Kearsey and Hyne, 1994), multiple regression apart, then an analysis of the phenotypic and marker data (Wright and Mowers, 1994; Whittaker et al., 1996), and with an appropriate statistical method as implemented in a composite interval mapping (Zeng, 1994). Association user-friendly software package will almost always lead to mapping, which requires collections of germplasm instead the identifi cation of at least a few markers associated with of biparental populations, has also been developed as a each trait of interest. In short, in the last 20 years we have method for fi nding genes underlying quantitative traits learned how to routinely map QTL. (Hästbacka et al., 1992; Lazzeroni, 1997; Yu et al., 2006). In contrast, exploiting the QTL that have been Software packages for mapping with F2 or backcross pop- mapped has not been routinely done. While the following ulations, selfed or recombinant-inbred progenies, or ger- statement may seem harsh [and I myself, with colleagues, mplasm collections include MAPMAKER/QTL (Lincoln have previously reported a total of 172 QTL in maize (Lu 1650 WWW.CROPS.ORG CROP SCIENCE, VOL. 48, SEPTEMBER–OCTOBER 2008 et al., 2003a,b; Parisseaux and Bernardo, 2004)], the vast stringency for declaring the presence of a QTL, require majority of the favorable alleles at these identifi ed QTL diff erent levels of resolution for pinpointing QTL loca- reside in journals on library shelves rather than in culti- tion, and usually require diff erent types of germplasm vars that have been improved through the introgression (Fig. 1). As shown later in this article, the most useful or selection of these favorable QTL alleles. This is cer- breeding procedures diff er between the two breeding sub- tainly not to say that there have been no examples of QTL goals.
Details
-
File Typepdf
-
Upload Time-
-
Content LanguagesEnglish
-
Upload UserAnonymous/Not logged-in
-
File Pages16 Page
-
File Size-