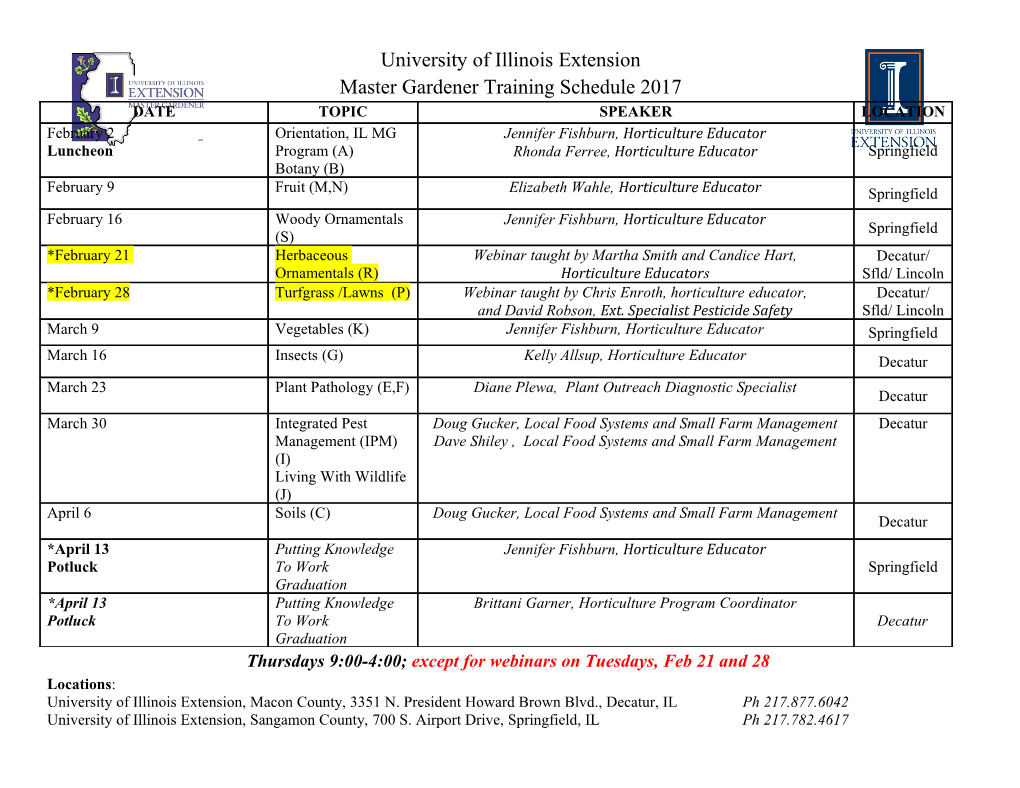
Anatomy and Pathology/Oncology Selective Equatorial Sclera Crosslinking in the Orbit Using a Metal-Coated Polymer Waveguide Sheldon J. J. Kwok,1,2 Sarah Forward,1 Christian M. Wertheimer,1 Andreas C. Liapis,1 Harvey H. Lin,1 Moonseok Kim,1 Theo G. Seiler,1,3,4 Reginald Birngruber,1,5 Irene E. Kochevar,1 Theo Seiler,3 and Seok-Hyun Yun1,2 1Harvard Medical School and Wellman Center for Photomedicine, Massachusetts General Hospital, Boston, Massachusetts, United States 2Harvard-MIT Health Sciences and Technology, Massachusetts Institute of Technology, Cambridge, Massachusetts, United States 3Institute for Refractive and Ophthalmic Surgery (IROC), Zurich, Switzerland. 4Universit¨atsklinik fur¨ Augenheilkunde, Inselspital, Universit¨at Bern, Bern, Switzerland 5Institut fur¨ Biomedizinische Optik, Universit¨at zu Lubeck,¨ Lubeck,¨ Germany Correspondence: Seok-Hyun Yun, PURPOSE. Photochemical crosslinking of the sclera is an emerging technique that may prevent Harvard University, 65 Landsdowne excessive eye elongation in pathologic myopia by stiffening the scleral tissue. To overcome St. UP-525, Cambridge, MA 02139, the challenge of uniform light delivery in an anatomically restricted space, we previously USA; introduced the use of flexible polymer waveguides. We presently demonstrate advanced [email protected]. waveguides that are optimized to deliver light selectively to equatorial sclera in the intact SJJK and SF contributed equally to the orbit. work presented here and should therefore be regarded as equivalent METHODS. Our waveguides consist of a polydimethylsiloxane cladding and a polyurethane authors. core, coupled to an optical fiber. A reflective silver coating deposited on the top and side surfaces of the waveguide prevents light leakage to nontarget, periorbital tissue. Postmortem Submitted: January 22, 2019 Accepted: May 15, 2019 rabbits were used to test the feasibility of in situ equatorial sclera crosslinking. Tensometry measurements were performed on ex vivo rabbit eyes to confirm a biomechanical stiffening Citation: Kwok SJJ, Forward S, Wert- effect. heimer CM, et al. Selective equatorial sclera crosslinking in the orbit using a RESULTS. Metal-coated waveguides enabled efficient light delivery to the entire circumference metal-coated polymer waveguide. In- of the equatorial sclera with minimal light leakage to the periorbital tissues. Blue light was vest Ophthalmol Vis Sci. delivered to the intact orbit with a coefficient of variation in intensity of 22%, resulting in a 45 2019;60:2563–2570. https://doi.org/ 6 11% bleaching of riboflavin fluorescence. A 2-fold increase in the Young’s modulus at 5% 10.1167/iovs.19-26709 strain (increase of 92% P < 0.05, at 25 J/cm2) was achieved for ex vivo crosslinked eyes. CONCLUSIONS. Flexible polymer waveguides with reflective, biocompatible surfaces are useful for sclera crosslinking to achieve targeted light delivery. We anticipate that our demonstrated procedure will be applicable to sclera crosslinking in live animal models and, potentially, humans in vivo. Keywords: sclera, waveguide, crosslinking, myopia espite the rapidly increasing prevalence of myopia cornea, the sclera cannot be irradiated easily using an external D worldwide, interventions to control myopia progression light source. In designing a light-delivery apparatus to deliver have been inadequate. Of particular concern are patients with light in the orbit, selective and uniform illumination also are high myopia, who have an increased risk for potentially sight- critical requirements.16,19–22 threatening diseases, such as glaucoma and retinal detach- SXL using riboflavin in conjunction with ultraviolet A (UVA) ment.1 Various therapeutic modalities have been tested to halt or blue light has shown efficacy in reducing axial length myopic progression and prevent these complications.2,3 elongation in several animal models of myopia,7–9 primarily The sclera has an important role in the development and targeting the scleral equator. Although protrusion of the progression of myopia.4,5 Scleral remodeling in myopia includes posterior sclera is a hallmark of severe pathologic myopia,6 scleral thinning, loss of scleral tissue, weakening of the scleral and while the whole sclera and cornea also show pathogno- mechanical properties and, ultimately, development of poste- monic changes in myopia progression,4 targeting the equator rior staphyloma.6 This has led to the development of scleral has several advantages. The equator is relatively easy to access collagen crosslinking (SXL) with light-activated photosensitizers surgically,andfurthermore,anypotentialphotochemical to mechanically reinforce the scleral tissue and prevent toxicity would not be as severe as it would be at the posterior subsequent changes in axial length.7–16 Corneal collagen pole or the limbus, where nearby essential anatomic structures crosslinking with light-activated photosensitizers currently is can be irreversibly damaged. used clinically to treat patients with corneal ectatic disor- To target the equatorial region, fiberoptic approaches have ders.17,18 However, significant technical challenges prevent been used to target small, selected regions of the sclera7,9 (<20 clinical translation of SXL for myopia control. Compared to the mm2). In another study, a dental blue light source was used to Copyright 2019 The Authors iovs.arvojournals.org j ISSN: 1552-5783 2563 This work is licensed under a Creative Commons Attribution-NonCommercial-NoDerivatives 4.0 International License. Metal-Coated Polymer Waveguides for Scleral Crosslinking IOVS j June 2019 j Vol. 60 j No. 7 j 2564 contact the exposed sclera to irradiate a relatively larger area8,23 (~100 mm2). Active devices, such as miniature light- emitting diodes (LEDs) also can be used but have several drawbacks, including limited irradiance, risk of thermal tissue damage, and poor flexibility.20,24 Recently, we introduced the use of thin and flexible polymer waveguides for light delivery to the sclera, and demonstrated SXL of equatorial sclera around the entire circumference (~400 mm2) of an ex vivo porcine eyeball. Compared to other devices, our waveguides enable uniform light delivery to large areas of the sclera with shorter procedural times and reduced invasiveness.16 We described a novel waveguide design optimized for SXL in vivo. Our waveguides have two major advantages over previous approaches. First, we use a core-cladded structure to ensure efficient light propagation irrespective of the surround- ing environment, enabling light delivery inside the orbit while the waveguide is in contact with extraocular muscles, fluid, and other tissues. Second, a reflective metal coating deposited around the waveguide blocks light leakage from the outer surface of the waveguide, minimizing off-target irradiation to surrounding tissues, and decreasing risk of photochemical toxicity. The reflective coating also reduces optical loss in the waveguide when the waveguide is curved around the eyeball. As a proof of principle, we used our waveguides to irradiate equatorial sclera in a freshly excised rabbit head. We reported FIGURE 1. Schematic of a waveguide inserted in the orbit for the surgical procedure to insert the waveguide into the orbit, crosslinking of equatorial sclera. and successfully demonstrated photobleaching of riboflavin around the eye. Finally, we confirmed stiffening of equatorial For metal coating, the polymer waveguides were mounted sclera with our technique on ex vivo rabbit eyes. on a carrier wafer and placed in a sputter coater (208HR; Cressington, Watford, United Kingdom) such that the strips were off-angle with respect to the sputtering source. Eight METHODS sequential depositions of approximately 15 nm of silver were Intended Use of Waveguides performed, manually varying the angle of the samples within the chamber after each one. As a result of this procedure, all Our waveguides were designed to be inserted into the Tenon’s sides other than the therapeutic side received a uniform space underneath the rectus muscles within the orbit (Fig. 1). coating of metal. To protect the silver deposition, a thin layer of To enable insertion into such a tight anatomic space, we <1 mm PDMS was applied over the top. The PDMS outer layer selected materials with high mechanical flexibility. For optimal ensures good biocompatibility with the tissue in contact. light delivery, we also chose materials with high optical To couple light into the waveguides, a 200 lm core ferrule transparency. The optical design of the waveguide was patch cable (M95L01, 0.50 numerical aperture (NA), Ø1.25 mm, optimized to deliver light from one side to scleral tissue, while Thorlabs, Inc., Newton, NJ, USA) was connected to a 5 mm minimizing light leakage to all other surfaces, preventing light long, 200 lm core fiberoptic cannula (CFML52L05, 0.50 NA, exposure of extraocular muscles and other periorbital tissues. Ø1.25 mm; Thorlabs, Inc.) via quick release interconnect Light was coupled into the waveguide through a laser-coupled (ADAL3; Thorlabs, Inc.). The cannula was inserted into the pigtail fiber, allowing convenient selection of wavelength and core on the short edge of the waveguide. A 30-gauge needle was incident power. used to perforate the core for easier insertion. Images of blue light coupled into the waveguide were taken from the top, Waveguide Fabrication bottom, and side to visualize the effect of the metal coating. Tapered PDMS-only waveguides were fabricated for com- Polydimethylsiloxane (PDMS; Sylgard 184; Dow Corning, parison following
Details
-
File Typepdf
-
Upload Time-
-
Content LanguagesEnglish
-
Upload UserAnonymous/Not logged-in
-
File Pages8 Page
-
File Size-