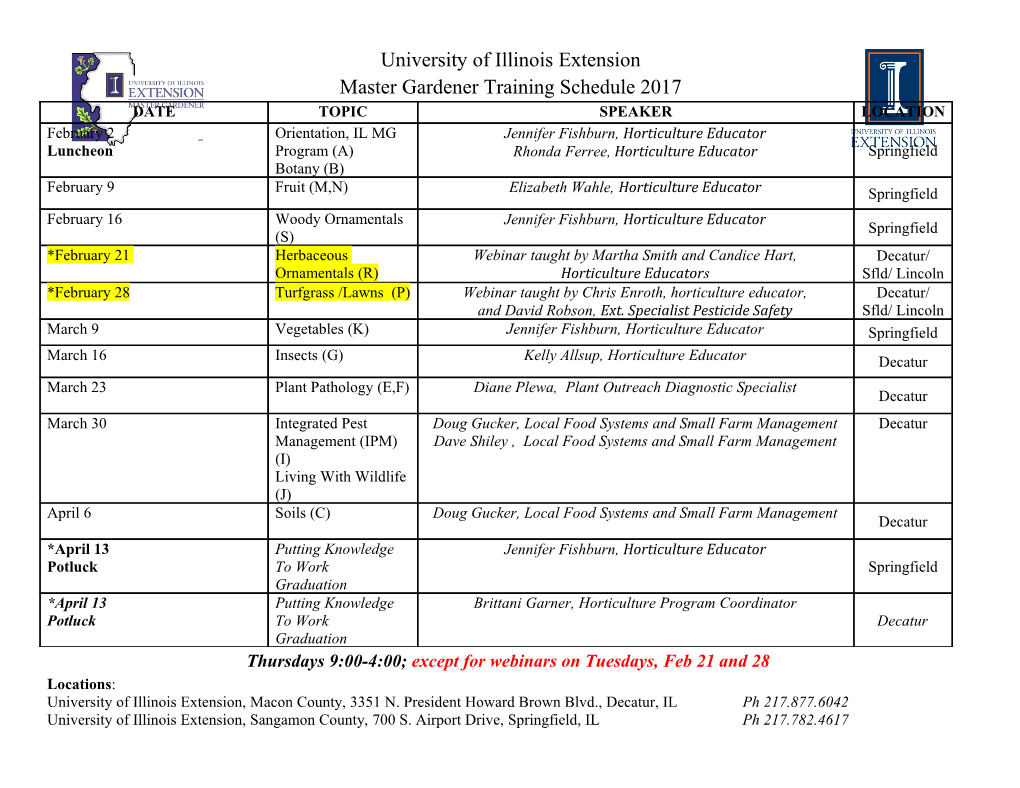
Journal of Fish Biology (2017) 91, 460–472 doi:10.1111/jfb.13351, available online at wileyonlinelibrary.com Teeth penetration force of the tiger shark Galeocerdo cuvier and sandbar shark Carcharhinus plumbeus J. N. Bergman*†‡, M. J. Lajeunesse* and P. J. Motta* *University of South Florida, Department of Integrative Biology, 4202 East Fowler Avenue, Tampa, FL 33620, U.S.A. and †Florida Fish and Wildlife Conservation Commission, Florida Fish and Wildlife Research Institute, 100 Eighth Avenue S.E., Saint Petersburg, FL 33701, U.S.A. (Received 16 February 2017, Accepted 15 May 2017) This study examined the minimum force required of functional teeth and replacement teeth in the tiger shark Galeocerdo cuvier and the sandbar shark Carcharhinus plumbeus to penetrate the scales and muscle of sheepshead Archosargus probatocephalus and pigfish Orthopristis chrysoptera. Penetra- tion force ranged from 7·7–41·9and3·2–26·3 N to penetrate A. probatocephalus and O. chrysoptera, respectively. Replacement teeth required significantly less force to penetrate O. chrysoptera for both shark species, most probably due to microscopic wear of the tooth surfaces supporting the theory shark teeth are replaced regularly to ensure sharp teeth that are efficient for prey capture. © 2017 The Fisheries Society of the British Isles Key words: biomechanics; bite force; Elasmobranchii; teleost; tooth morphology. INTRODUCTION Research on the functional morphology of feeding in sharks has typically focused on the kinematics and mechanics of cranial movement (Ferry-Graham, 1998; Wilga et al., 2001; Motta, 2004; Huber et al., 2005; Motta et al., 2008), often neglecting to integrate the function of teeth (but see Ramsay & Wilga, 2007; Dean et al., 2008; Whitenack et al., 2011). While some studies have examined the design and performance of shark teeth during puncture and draw (Frazzetta, 1988; Lucifora et al., 2001; Whitenack & Motta, 2010; Whitenack et al., 2011; Corn et al., 2016), a further exploration in the complexity of shark tooth structure is essential in elucidating the biological role that teeth play in feeding and particularly during successful prey capture. Extant fishes have distinct tooth morphologies that appear related totheir species-specific feeding behaviours (Cappetta, 1987; Wainwright & Richard, 1995; Kajiura & Tricas, 1996; Ramsay & Wilga, 2007; Whitenack & Motta, 2010). Stem- ming from these various tooth morphologies and accompanying diets, there has been a tendency to classify shark teeth into clutching, puncturing, slicing, crushing and grinding ecomorphological types (Moss, 1977; Cappetta, 1987; Frazzetta, 1988). For example, the broad, serrated teeth found in the white shark Carcharodon carcharias (L. 1758) have been suggested as a characteristic of species that gouge chunks of flesh ‡Author to whom correspondence should be addressed. Tel.: +1 904 5536508; email: [email protected] 460 © 2017 The Fisheries Society of the British Isles SHARK TOOTH PENETRATION FORCE 461 from large, soft-prey items, whereas the long, pointed teeth in the sand tiger shark Carcharias taurus Rafinesque 1810 exemplify a piscivorous diet that pierce and grasp fast moving fishes (Frazzetta, 1988; Lucifora et al., 2001; Smale, 2005; Ferrara et al., 2011). Dentitions may also be suited to the stress experienced during feeding: long, pointed teeth for soft-bodied and slippery prey, contrasted to broad, serrated teeth for hard, dense prey (Frazzetta, 1988; Whitenack & Motta, 2010; Motta & Huber, 2012). In contrast with the historical tendency to group tooth shapes into biomechanical roles, Whitenack & Motta (2010) found that puncturing performance (force to punc- ture) differed among different prey items, indicating that not all soft prey are alike, but there were few patterns that distinctly categorized tooth performance based on their shape. Material testing of shark teeth during puncturing and cutting (draw) on a variety of hard and soft prey established the majority of teeth were able to puncture different prey items, with broader, triangular tooth types being somewhat less effective at punc- ture than narrow-cusped teeth. Broad teeth, such as those of the tiger shark Galeocerdo cuvier (Péron & LeSueur 1822), often failed in puncture of hard and soft prey, simply crushing the prey when driven directly down into it. Their study revealed force to punc- ture bony fishes was remarkably low, ranging from <1 to 67 N. Force to puncture in bony fish teeth have been found in low ranges from <1 to 2 N (Galloway et al., 2016). Low forces for puncture stand in contrast to relatively high bite forces found in many sharks. For instance, the bite force of C. carcharias, the bull shark Carcharhinus leucas (Valenciennes 1839) and the great hammerhead shark Sphyrna mokarran (Rüppell 1837) place among the highest of all extant vertebrates and produce a considerably higher bite force than what is required for puncture (Habegger et al., 2012). Durophagous species (hard-prey consumers) generate particularly high bite forces suggesting an evolutionary strategy for processing hard-prey items. Conversely, jaw closing mechanisms in piscivorous sharks may be more speed efficient rather than force efficient, as in the blacktip shark Carcharhinus limbatus (Valenciennes 1839) and geared towards capturing soft-prey items (Huber et al., 2005; Whitenack & Motta, 2010). Consistent with the bite forces of large sharks, a finite element analysis of shark teeth indicated they are structurally strong and suited for a suite of prey capture strategies (Whitenack et al., 2011). Fossil records suggest the earliest shark teeth evolved from dermal denticles that lined the mouth and with an increase in dietary diversity, elasmobranchs encompassed a variety of tooth sizes and shapes to accommodate more heterogeneous prey options (Zangerl, 1981; Motta & Huber, 2012). As diet progressed to include increasingly larger prey, the ancestral slow tooth replacement condition was lost and evolved to synchronous, rapid tooth replacement by moving newer teeth lingually in waves to serve as functional teeth (Motta & Huber, 2012; Rasch et al., 2016). The evolutionary combination of structurally strong, polyphyodont dentition combined with high bite force suggests the frequency of tooth replacement in sharks is driven by tooth wear, not tooth failure (Whitenack et al., 2011; Motta & Huber, 2012; Corn et al., 2016), an idea also supported by dynamic testing of shark teeth (Corn et al., 2016). Conse- quently, replacement teeth should be sharper and require less force to penetrate prey than worn, functional teeth. To define feeding processes and behaviour in sharks, determining how different tooth morphotypes function, including force to penetration, is critical in understanding prey capture and processing. Therefore, this study investigated the minimum penetra- tion force of two shark species with dissimilar tooth morphologies [the sandbar shark © 2017 The Fisheries Society of the British Isles, Journal of Fish Biology 2017, 91, 460–472 462 J. N. BERGMAN ET AL. Carcharhinus plumbeus (Nardo 1827) and G. cuvier], during penetration of teleost prey with large and small elasmoid scales [sheepshead Archosargus probatocephalus (Wal- baum 1792) and pigfish Orthopristis chrysoptera (L. 1766)]. This study also seeks to determine if older, functional teeth require more force to penetrate prey than unused and presumably sharper replacement teeth. For analysis purposes, functional and replace- ment teeth have been categorized as tooth types. Penetration is defined here as the initial piercing of the prey by the tooth tip, whereas puncture might involve further excursion of the tooth into the prey requiring more force as the surface area of the tooth increases. MATERIALS AND METHODS SPECIMENS FOR PENETRATION TESTING Teeth from the functional row and first replacement row of C. plumbeus and G. cuvier were glued to a cantilever beam and driven into the epaxial muscle below the dorsal fin of two species of bony fishes, A. probatocephalus [n = 6, mean range standard length (LS) 25–30 mm], with larger elasmoid scales (n = 20, mean ± s.d. thickness and diameter 0·2 ± 0·0mm and 10·2 ± 0·7 mm, respectively) and O. chrysoptera (n = 6, mean range LS 15–20 mm), with smaller elasmoid scales (n = 20, mean ± s.d. thickness and diameter 0·1 ± 0·02 mm and 3·4 ± 0·3 mm, respectively). Additionally, scale overlap was determined from two specimens of each species by marking and removing scales from the test area and measuring the overlap with a digital micrometre and expressed as per cent overlap. For A. probatocephalus and O. chrysoptera the scales of the epaxial region overlapped by 50 and 52%, with 50 and 48% of the posterior edge of the scale protruding beyond the scale immediately anterior to it, respectively. One jaw from each shark species was used in this study (n = 2). Three functional and three immediately posterior replacement teeth were removed from the lower jaw (Lowry et al., 2009). Lower-jaw teeth were utilized as these teeth generally are more pointed in carcharhiniform sharks and impale the prey first, whereas the triangular upper teeth saw through the prey during lateral head shaking (Frazzetta & Prange, 1987; Compagno, 1988; Frazzetta, 1988; Smale et al., 1995; Motta & Huber, 2012). Each tooth underwent three penetration trials first on O. chrysoptera and then on A. probatocephalus to reduce any possible dulling of teeth, for a total of 72 trials. Each teleost endured a maximum of nine penetrations per epaxial region, such that penetration holes did not overlap. Teleost specimens were frozen within 1 h of landing and thawed in cool water for 1 h prior to testing. Trials were conducted on each teleost for no >1h after thawing. The shark jaws and attached teeth were provided from Gulf of Mexico waters by recreational and commercial fishers. The fishers, however, neglected to include total length (LT), so applying inter-tooth distance methods according to Lowry et al. (2009), the estimated LT range of the sharks C. plumbeus and G. cuvier were 2027–2074 and 1998–2048 mm, respectively. PENETRATION TESTING Each tooth was fixed with epoxy glue to a removable 27·3 mm diameter PVC coupling (Fig.
Details
-
File Typepdf
-
Upload Time-
-
Content LanguagesEnglish
-
Upload UserAnonymous/Not logged-in
-
File Pages13 Page
-
File Size-