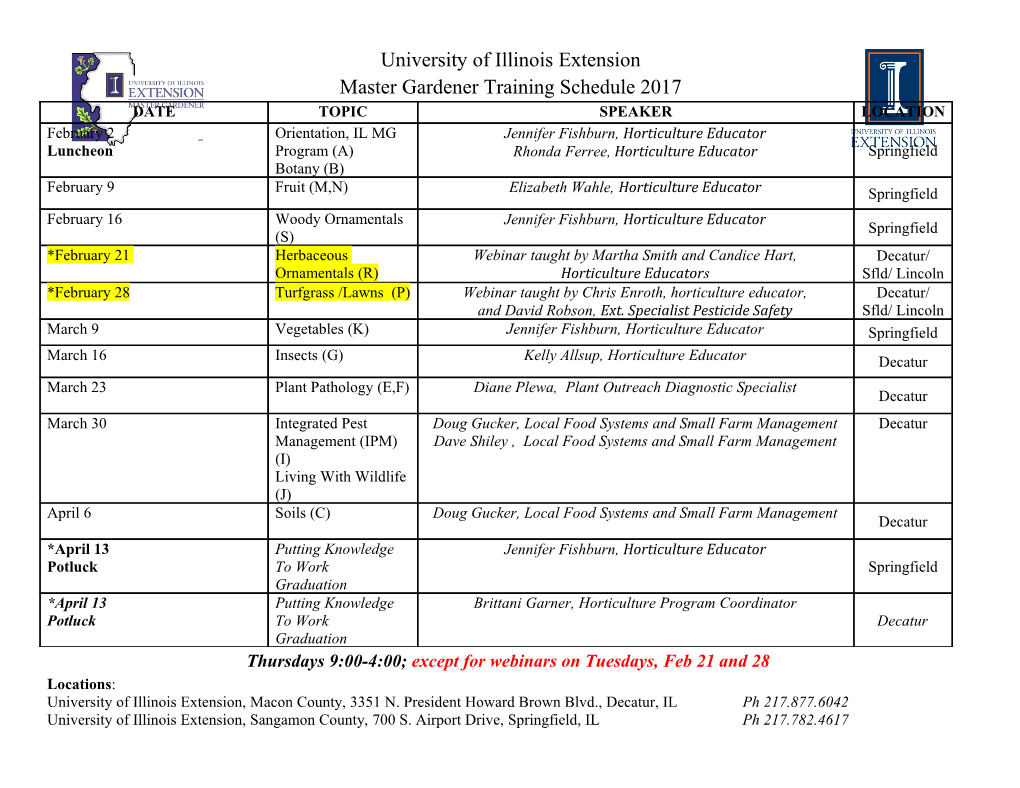
J. Cell Set. 44, 395-435 (1980) 295 Printed in Great Britain © Company of BiologitU Limited igSo NUCLEAR NON-CHROMATIN PROTEINACEOUS STRUCTURES: THEIR ROLE IN THE ORGANIZATION AND FUNCTION OF THE INTERPHASE NUCLEUS PAUL S. AGUTTER* AND JONATHAN C. W. RICHARDSONf • Department of Biological Sciences, Napier College, Colinton Road, Edinburgh EH10 5DT, Scotland and f Department of Physiology and Pharmacology, University of St Andrews, Bute Medical Buildings, St Andrews, Fife, Scotland REVIEW ARTICLE: CONTENTS I. INTRODUCTION page 39s (1) Historical background 395 (2) Nomenclature 397 II. NUCLEAR PROTEIN MATRIX AND NUCLEAR GHOSTS 397 (1) Isolation 397 (2) Composition 398 (3) Ultrastructure 401 (4) Enzyme activities associated with the nuclear protein matrix 405 (5) Contractility of the nuclear protein matrix 405 (6) Functions associated with the nucleai protein matrix 408 III. SUBFRACTIONS OF THE NUCLEAR PROTEIN MATRIX 411 (A) The pore-lamina 411 (1) Isolation 411 (2) Composition 413 (3) Ultrastructure 414 (4) The molecular organization of the pore-lamina 417 (B) Other subfractions 419 IV. COMPOSITIONAL AND FUNCTIONAL DIFFERENCES BETWEEN THE PORE-LAMINA AND THE REMAINDER OF THE NUCLEAR PROTEIN MATRIX 42O V. PROSPECTS FOR FURTHER RESEARCH 422 (1) The role of the nuclear protein matrix in nucleo-cytoplasmic RNA transport 422 (2) Relevance of a knowledge of factors affecting the stability of the intra- nuclear regions of the matrix to the further development of methods for isolation of the nuclear envelope 423 (3) Fate of the nuclear matrix during mitosis 423 I. INTRODUCTION (1) Historical background Since 1949 there have been several accounts of a 'honeycomb layer' or 'nuclear cortex' in the nuclei of lower eukaryotes (Callan, Randall & Tomlin, 1949; Callan & Tomlin, 1950; Harris & James, 1952; Pappas, 1956; Beams, Tahmisian, Devine & 26-2 396 P. S. Agutter andj. C. W. Richardson Anderson, 1957; Mercer, 1959; Gray & Guillery, 1963; Daniels & Breyer, 1967; Barton, Kisieleski, Wassermann & Mackevicius, 1971). This highly structured layer appears either to separate the inner nuclear membrane from the peripheral chromatin or to structure the heterochromatin region. It extends for up to 300 nm into the nucleoplasm, though this distance varies considerably with cell type, and shows discontinuities in the region of the pore complexes. In the decade 1960-70 a similar, but less extensive highly organized layer of material was described in the nuclei of higher eukaryotes. The structure was termed, alternatively, the 'granular perinuclear layer' (Bruni & Porter, 1965), 'dense lamella' (Kalifat, Bouteille & Delarue, 1967), 'fibrous lamina' (Coggeshall & Fawcett, 1964; Fawcett, 1966; Ghadially, Bhatnager & Fuller, 1972; Cohen & Sundeen, 1976) or 'zonula nucleum limitans' (Mazanek, 1967; Patrizi & Poger, 1967). Davies and his co-workers, in a detailed study of this layer, found that it comprised alternating narrow electron-dense and electron-transparent bands arranged parallel to the envelope, intersected orthogonally by fine electron-dense fibrils (Davies, 1967, 1968; Davies & Small, 1968). Detailed investigation of amphibian oocyte nuclear envelopes revealed, instead of this perinuclear layer, an array of fibres extending from the nuclear face of the pore complexes into the nucleoplasm (Franke & Scheer, 1970). Collectively, these studies suggested that at least some part of the region of the nucleus contiguous with the inner nuclear membrane is structured by a network of fibrils continuous with the pore complexes. Such a structure has since been isolated and termed the 'nuclear pore-lamina fraction' (Aaronson & Blobel, 1974, 1975). Over the years 1942-69, evidence accrued for the existence of a structural network of non-chromatin protein fibrils extending throughout the nucleus (Mayer & Gulick, 1942; Zbarsky & Debov, 1948; Wang et al. 1950; Allfrey, Dally & Mirsky, 1955; Du Praw, 1956; Zbarsky & Georgiev, 1959; Georgiev & Chentsov, 1962; Zbarsky, Dmitrieva & Yermolayeva, 1962; Wang, 1961, 1966; Steel&Busch, 1963; Bernhard & Granbonlan, 1963; Swift, 1963; Holtzman, Smith & Penman, 1966; Kaye & McMaster-Kaye, 1966; Monneron & Bernhard, 1969). Such a network has since been isolated (Berezney & Coffey, 1974a, b) and termed the 'nuclear protein matrix'. The early evidence for the structure depended on its resistance to the procedures used for the solubilization of chromatin: it retained the original shape of the nucleus after extraction with 2 M NaCl and dilute alkali. Corroborating evidence included: (a) the observation that while nuclease treatment did not markedly alter the shape and size of the nucleus, protease treatment quickly resulted in swelling and rupture (Anderson, 1953); and (b) the maintenance of the gross morphology of the nucleus after removal of the nuclear membranes with non-ionic detergents (Bach & Johnston, 1967). Later investigations by Busch and co-workers led to the idea of a structural ribonucleo- protein network, rather than a merely proteinaceous structure, extending throughout the nucleus (Smetana, Steele & Busch, 1963; Steele & Busch, 1966; Narayan, Steele, Smetana & Busch, 1967). Before 1970, therefore, there was a substantial body of literature concerning at least 2 sorts of non-membranous structural elements inside nuclei. It seems reasonable a priori to regard the juxtamembranous layer described in detail by Davies (1968) as Nuclear matrix, ghost and pore-lamina 397 the peripheral portion of the protein or ribonucleoprotein network discussed above. Since 1970, several biochemical studies of these structural elements have been carried out. The present review is devoted to the implications of these studies for an under- standing of the organization of nuclei and their functions during interphase. (2) Nomenclature Before discussion of these studies can be undertaken, clarification of the terminology is essential. Different laboratories naturally develop different systems of nomenclature; the system outlined here is based, as far as possible, on the most frequent current usage. It should be emphasized that our terminology does not distinguish between a structure observed in situ by microscopy and an apparently similar but not necessarily identical structure prepared by subfractionation of isolated nuclei. To make such a distinction would greatly complicate the nomenclature, but its importance in the interpretation of experimental findings should not be overlooked. We use the term nuclear envelope to describe the peripheral structure isolated from nuclei. The nuclear envelope has 4 ultrastructurally distinct subfractions: outer nuclear membrane, inner nuclear membrane, pore complexes and fibrous lamina. Removal of the 2 membranes leaves the ' nuclear pore-lamina fraction' or, more briefly, pore-lamina (see above and section in A, below). Careful removal of the chromatin and the 2 nuclear membranes from whole nuclei leaves a structure which has been called the nuclear protein matrix (see above and section 11, below). This structure includes the pore-lamina: pore complexes inter- connected with fibrils are visible in it. If nuclei are vigorously extracted with deter- gent and high-ionic-strength media but not treated with nucleases, nuclear ghosts can be banded out on sucrose gradients. The distinction between these nuclear ghosts (which are operationally distinct from nuclear envelopes) and the nuclear protein matrix is discussed in section 11. The nuclear protein matrix appears to contain residual nucleoli as morphologically distinct regions. II. NUCLEAR PROTEIN MATRICES AND NUCLEAR GHOSTS (1) Isolation The nuclear protein matrix. Berezney, Coffey and co-workers have evolved a pro- cedure for isolating nuclear protein matrix from whole nuclei (Berezney & Coffey 1974a, b, 1975a, b, 1977). Essentially, the method involves: (a) swelling of the nuclei (isolated by a variant of the method of Blobel & Potter, 1966) by overnight storage at 5 °C and treatment with a buffer containing a low concentration (0-2 ITIM) of mag- nesium ions; (b) solubilization of the chromatin in 2 M NaCl; (c) removal of the nuclear membranes with 1 % (w/v) Triton X-100; and (d) removal of residual nucleic acids with DNase and RNase. More recently, a modified procedure involving omission of step (a) and inclusion of inhibitors of proteolysis in the media has been employed. This modified procedure has been claimed to result in the production of more intact matrices (Berezney & Buchholtz, 1978). This or closely related methods for isolating nuclear protein matrices have been successfully used in other laboratories (Shelton, 398 P. S. Agutter andj. C. W. Richardson Cobbs, Povlishock & Burkat, 1976; Faiferman & Pogo, 1975; Hildebrand, Okinaka & Gurley, 1975; Hodge, Mancini, Davis & Heywood, 1977; Agutter & Birchall, 1979). Faiferman & Pogo (1975) have also isolated the matrix fraction, albeit in a fragmented state, from nuclei disrupted by nitrogen cavitation or in a French pressure cell. The nuclear ghost. Riley, Keller and co-workers have described the isolation of nuclear ghosts by a procedure involving: (a) washing of the nuclei in 1 % Tween 40 and 0-5% (w/v) sodium deoxycholate; (b) disruption of the nuclear contents with 0-5 M MgCl2; and (c) sucrose gradient centrifugation. The nuclear ghosts band on the gradient at 47-52% (w/v) sucrose (Riley, Keller & Byers, 1975; Riley & Keller, 1976a, b, 1978a, b; Keller & Riley, 19760, b). (2) Composition The nuclear protein matrix. The nuclear protein matrix accounts for about 10-20% of the total nuclear
Details
-
File Typepdf
-
Upload Time-
-
Content LanguagesEnglish
-
Upload UserAnonymous/Not logged-in
-
File Pages42 Page
-
File Size-