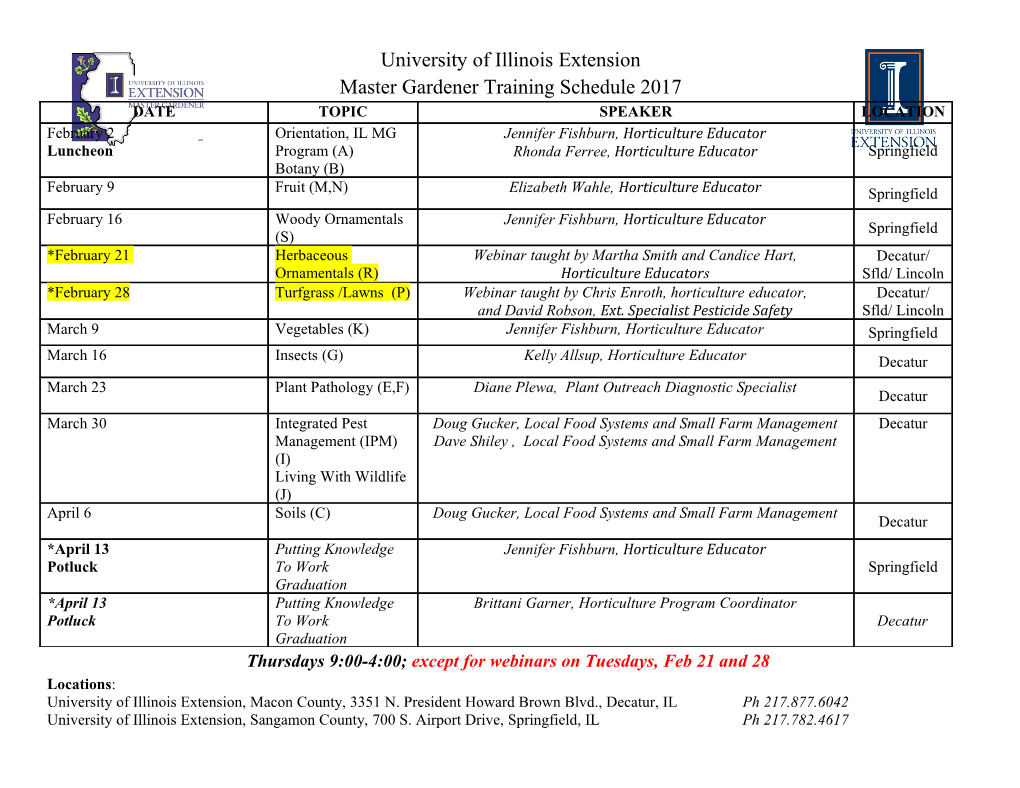
41st Lunar and Planetary Science Conference (2010) 1171.pdf MESOSCALE SIMULATION OF ATMOSPHERIC RESPONSE TO CHAOS TERRAIN FORMATION. E.S. Kite1, S.C.R. Rafkin2, T.I. Michaels2, M. Manga1. 1Earth and Planetary Science, University of California, Ber- keley ([email protected]), 2 Department of Space Studies, Southwest Research Institute, Boulder, Colorado. Introduction: Water ponded within Juventae and Model output: Condensation of vapor released by Echus Chasmata during outflow channel formation [1]. the lake drives a circulation in the vertical plane with Unusually young channel networks are preserved on some similarities to tropical cyclones on Earth: strong plateaux to the W of both chasms [2-3], superimposed low-level winds converge in the south of the lake, on Late Hesperian basalts. The Juventae Chasma pla- leading to high water vapor mass fractions (up to 0.3) teau channel networks are preserved in inverted relief, near the surface. Maximum water ice mass fractions within light-toned layered deposits that contain opal (~1%) and vertical velocities (~50 m/s) occur ~30 km and hydroxylated ferric sulfate [4-5]. Cross-cutting above the lake surface. relationships imply that channel formation was epi- Water-ice precipitation on the chasm flanks has sodic, beginning before backwasting of the chasm two local maxima: on a promontory northwest of Ju- walls to their present position, but continuing after- ventae Chasma, and near the center of the south wall of wards [6]. Localized precipitation during chaos terrain Juventae Chasma. There is good spatial correspon- formation has been proposed to explain these channels dence (Figure 1) between the first local maximum and [7]. We report preliminary results from MRAMS the inverted channels and light-toned layered deposits (Mars Regional Atmospheric Modeling System) mapped with HiRISE [6]. However, there is no evi- mesoscale simulations intended to test this hypothesis. dence for channels or light-toned layered deposits in These preliminary results are consistent with the local- available (CTX) images of the south chasm wall. We ized-precipitation hypothesis, and suggest that storms find high precipitation rates are restricted to the imme- associated with chaos terrain formation may mobilize diate vicinity of the lake. Excluding snowfall back into sand and perhaps gravel, but not boulders. the lake (60% of total snow), 50% of the snow falls Model description: MRAMS is a regional mesos- within 75 km of the lake edge, and 95% within 200 cale model of the Martian atmosphere with non- km. The mapped layered deposits and inverted chan- hydrostatic, fully compressible dynamics and dust, nels [6] fall within 50 km of the lake edge. water ice, and CO2 ice aerosol microphysics [8-9]. Sensitivity tests show a trend of reduced vapor re- Liquid water aerosol is not included. In the runs re- lease with reduced grid spacing in z, so the modeled ported here, we used 4 nested grids with the innermost precipitation rates are probably overestimated. On the grid having a horizontal resolution of 8.33 km, and a plateau, this rate peaks at ~3 g/cm2/day. vertical resolution of 30 m at the surface. Surface layer Energy and water budgets: Net evaporation from fluxes of heat, momentum and moisture are parameter- the lake (0.9 g/m2/s) is approximately equal to the ac- ized using a Monin-Obukhov scheme. cumulation rate of water ice beyond the lake margins. Boundary conditions: We flooded Juventae Assuming a mixing depth of 100m, this corresponds to Chasma to -1000m, fixing lake surface temperature to evaporative cooling of ~0.5 K / day. To prevent freez- 278.15K. In these preliminary runs, lake surface ing of the lake surface, either energy must be delivered roughness was unchanged from the land value (z0 = from deeper parts of the lake (by wind-driven mixing 0.03m). Boundary conditions for MRAMS are from or convection), or warm, buoyant water must be con- “normal year” output from the NASA Ames GCM at tinuously supplied from fractures at the lake floor. Ex- Ls ~ 270°. To prevent lake surface saturation vapor isting models of chaos hydrology [1, 11] show dis- pressures greater than atmospheric pressure, we dou- charge rates that exceed our simulated evaporation bled atmospheric pressure throughout the simulation. rates for hours to months, depending on assumed aqui- Gullies at high elevations suggest that atmospheric fer permeability. The temperature of the released water pressure was modestly greater in the geologically re- will evolve during the chaos event and will depend on cent past [10]. Following a spin-up period without va- the aquifer depth, the geothermal gradient, and the por release from the lake, the model was run for an presence/absence of a triggering volcanic intrusion additional 1.25 days with vapor release from the lake. [12]. Alternatively, stresses during spillway breaching After a brief (< ½ day) adjustment period, the location and outflow channel formation could mechanically and rate of water-ice precipitation stabilized, although disrupt ice cover. there is some variation with time-of-day. Once water- Comparison with observed channels and layered ice reaches the ground, albedo is set to 0.7. deposits: We traced all inverted channels in HiRISE image PSP_003724_1755, obtaining a drainage density of 1.33 km-1. Following the method of [13], we calcu- 41st Lunar and Planetary Science Conference (2010) 1171.pdf late the necessary melt rate to initiate sediment trans- rains include Ganges Chasma and Echus Chasma: port as a function of friction factor and grain size (Fig- these are future modeling targets. ure 2). Mobilization of clasts greater than 1 HiRISE pixel in diameter requires melting rates much greater than the simulated precipitation rates. To date no HiRISE-resolvable clasts have been reported: any fu- ture detections would be a severe challenge to the lo- calized-precipitation hypothesis. MRAMS tracks the accumulation of dust as ice particle nuclei. Assuming silicate dust density, the dust accumulation rate is < 1 cm/yr. Observed light-toned layer thicknesses are ~1 m [6], so if each layer corre- sponds to one chaos event < 1 yr in duration, an addi- tional source of silicates is needed. That source could be pre-existing aeolian deposits unrelated to the chaos event, or volcanic ash. Figure 2. Minimum melting rate (mm/hr) to initiate sediment transport (calculated using equation 6 in [13]), for a single inverted channel in HiRISE image PSP_003724_1755. Parameters (how obtained) are S = 0.44° (MOLA), W = 8 – 11.25 m (HiRISE), A = 2.6 2 -2 km (HiRISE), g = 3.7 ms , τ*c = 0.05, and ρ’ = 2. The simulated peak precipitation rate is 1.3 mm/hr (thick solid line), although this may be an overestimate (see text). The gray band represents order-of-magnitude uncertainty in peak precipitation rate. Melt rates can exceed precipitation rates if seasonal cycles act as a capacitor. References: [1] Harrison K.P. & Grimm R.E. (2008) JGR, 113, doi:10.1029/2007JE002951. [2] Mangold, N., et Figure 1. Water-ice precipitation rate (mm/hr), over- al. (2004) Science, 305, 78-81. [3] Williams, R.M.E., et al lain on MOLA topography (white is high, black is low, (2005), LPSC XXXVI, Abstract #1173. [4] Milliken, R.E., et elevation ranges from +5 to -3 km). Pale blue shade al. (2008) Geology, 36, 847-850. [5] Bishop, J.L., et al. corresponds to Juventae Chasma flooded to -1000 m (2009) JGR, 114 doi:10.1029/ 2009JE003352, [6] Weitz, with water at 5°C. Contours at 0.2 mm/hr intervals. C.M., et al. Icarus, in press. [7] Mangold, N., et al. (2008) Red and purple shades northwest of the chasm corre- JGR, 113, doi:10.1029/2007JE002985. [8] Rafkin, S.C.R., et spond to inverted channels and light-toned layered al. (2001) Icarus, 151, 2282-256. [9] Michaels, T.I., & rd deposits, respectively [6]. Rafkin, S.C.R. (2008) 3 Int’l Workshop on The Mars At- mosphere, Abstract #9116. [10] Head, J.W., et al. (2008), Next steps: Desirable improvements include a dy- PNAS, 105, 13258-13263. [11] Andrews-Hanna, J.C., & namic lake surface roughness parameterization [15] Phillips, R.J. (2007) JGR, 112, doi:10.1029/2006JE002881. and self-consistent lake thermodynamics. At the mo- [12] McKenzie, D. & Nimmo F. (1999) Nature, 397, 231- ment, MRAMS treats water vapor as a trace gas (the 233. [13] Perron, J.T., et al. (2006) JGR, 111, doi:10.1029/ pressure and virtual temperature effects of vapor re- 2005JE002602. [14] Hecht, M.H. (2002) Icarus, 156, 373- lease are not included). Other channels/layered depos- 386. [15] § 7.2 in Pielke Sr., R.A. (2002) Mesoscale mete- nd its on the Valles Marineris plateau close to chaos ter- orological modeling, 2 edn. .
Details
-
File Typepdf
-
Upload Time-
-
Content LanguagesEnglish
-
Upload UserAnonymous/Not logged-in
-
File Pages2 Page
-
File Size-