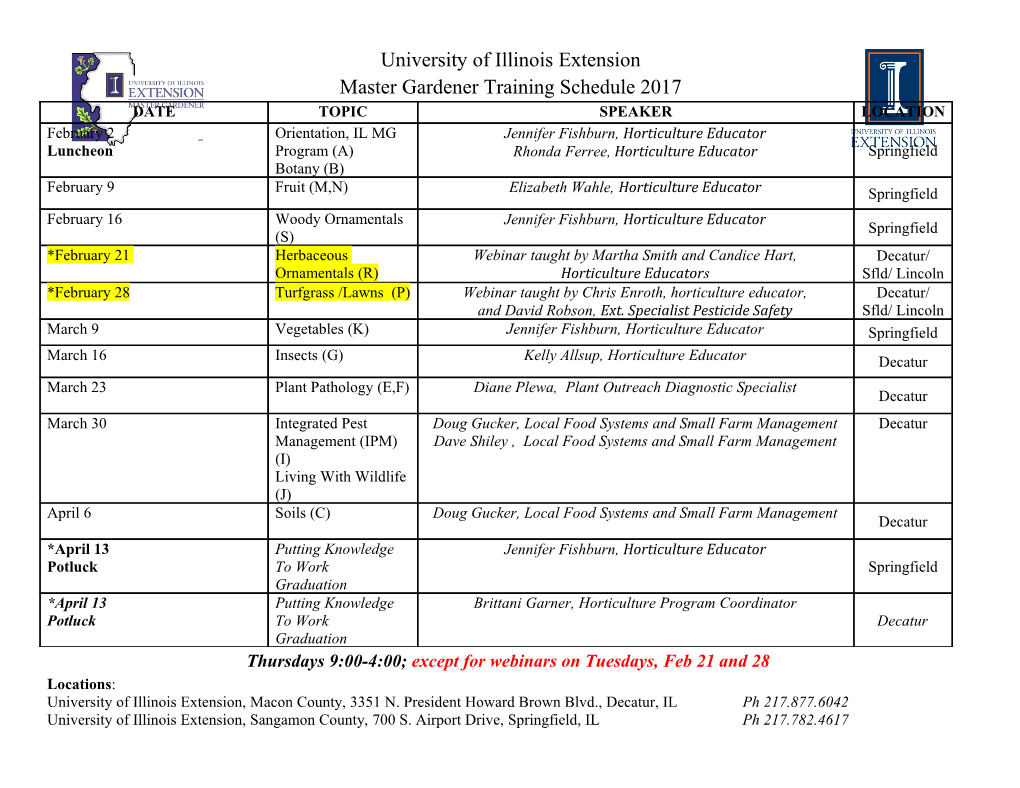
Breakthrough Starshot Bidder’s Briefing Phase 1 Photon Engine RFP October 2, 2017 Zoom Room BREAKTHROUGH INITIATIVES 1 2 3 4 5 6 © NRAO 7 © CSIRO ESO / DSS2 8 9 Breakthrough Starshot Pete Worden, Executive Director Pete Klupar, Project Manager STARSHOT ADVISORY COMMITTEE Avi Loeb, Harvard, Chairman Giacario Genta, Polytechnic Univ of Turin * Stephen Chu , Stanford Olivier Guyon, Univ of Arizona Saul Perlmutter*, Berkeley Mae Jemison, Astronaut, 100 Year Starship Freeman Dyson, Princetion Geoff Landis, NASA Glenn Ann Druyan Lord Martin Rees, Astronomer Royal Kelvin Long, J. British Interplanetary Soc. Ed Turner, Princeton Zac Manchester, Harvard Bruce Drain, Princeton Greg Matloff, NYC College of Technology Mason Peck, Cornell Kaya Nobuyuki, Kobe University Phil Lubin, UCSB Kevin Parkin, Parkin Research Jim Benford, µWave Sciences Bob Fugate, NM Tech (Emeritus) Lou Friedman, Planetary Society Mark Spencer, AFRL/RDL * Nobel Laureate Wesley Green, SETA 10 Starshot Objectives 1. Send a spacecraft to nearby stars with planets in the habitable zone within 5 Parsecs of earth 2. Collect Science Data of star system focused on planets and beam data back to Earth 3. Launch within 30 years, at an affordable cost 4. Go FAST! 11 The Alpha/Proxima Centauri star system is the principal objective for Starshot A planet, Proxima b, has been discovered in the habitable zone at Proxima Centauri 12 Solution to go fast 1. Lowest possible mass 2. Leave engine/fuel on Earth 3. Attach a chip to a sail 4. Laser beam is the wind StarChip - 0.22 gram 13 Long Term Schedule • $100M R&D over next 5 years to determine feasibility of Laser and Sail • Invest the value of the EELT from year 6 to year 11 build a low power prototype for space testing • Invest the value of the JWST from over 20 years for full scale laser system and space segment • First Proxima Nanocraft launch in ~30 years • 50 years from now first starchip arrives Proxima Centauri • 54.3 years from now first data received on Earth ESO concept of what Proxima Centauri might look like from the surface of Proxima b 14 Photon Engine R&D Schedule Three phases over 5 years • Phase 1 (this RFP) Phasing Concepts and Analysis, MOPA study • Phase 2 lab hardware validation demos, phasing and MOPA • Phase 3 phasing field demos, high power long coherence MOPA Up to • 11 awards Phase 1 • 5 awards Phase 2 • 3 awards Phase 3 15 16 Robert Forward, 1984 “The purpose of this paper is to show that interstellar flight by laser-pushed lightsails is not forbidden by the laws of physics. Whether it can be engineered and is We have arrived at those future financially or politically feasible is left for generations. future generations to determine.” Robert Fugate, 2017 Robert Forward, 1984. 17 Recent technology trends Miniaturization Nanotechnology Fiber Laser of electronics Material Sciences Technology Amplifiers Individual telescopes Phased at sail Phased Array Technology 18 So, how big does the laser need to be? Short answer: 50-100 GW and 1-5 km aperture 19 Concept Scenario Payload: 0.5 g Sail mass: 0.5 g Sail size: 3.78 m square Total mass: 1 g Sail thickness: 50 nm Laser power at sail: 35 GW Sail Density: 0.7 g/cm3 Flus on sail: 2.45 GW/m2 Sail reflectivity: 0.99995 Peak accel: 2.33e+5 m/s2 Sail Absorptivity: 0.001 Accel time: 247 sec Sail emissivity: 0.5 Final speed: 0.192 c Laser array size: 4km square Kinetic energy: 1.6e+12 J Total optical power: 70 GW Sail equil temp: 216K Beam efficiency: 0.5 Laser comm flux: 8.2e-4 ph s-1m-2 20 The constraints are: Speed: 0.2 c 1064 nm wavelength 60 Mm initial range Input parameters are: Cost of lasers $/W Cost of optics $/m2 Cost of power, energy storage $/kWh Sail parameters System Model Minimizes Photon Engine aperture to reach final speed Aperture vs transmit power trade to minimize cost 50% wall plug efficiency 21 System Level Design Example Circular Dielectric Sail –Reconverged 16 April 2017 Constraints Results 1.064 micron wavelength $12.6B CAPEX comprised of: 0.2 c target speed $5.3B lasers 60,000 km initial sail displacement from laser source $3.9B optics $3.3B energy storage Sail 1 g payload 3.0 m sail diameter (for minimum cost) 0.2 g/m2 areal density 2.4 g sail mass 0.001% absorptance 70% reflectance 53 GW transmitted power 970 K maximum temperature 1,115 sec (19 min) pulse length 1.6 effective emissivity (2-sided) 33 GWh stored pulse energy Beam Director 10,600 g’s initial acceleration (60,000 km from source) 0.1 $/Watt laser cost 800 g’s final acceleration (at 0.3 au from source) 100 $/m2 aperture cost 100 $/kWh storage cost 7.6 GW/m2 initial average beam intensity over sail Beamer minimum diameter such that final speed is 36 Pa initial photon pressure reachable. 249 N initial photon force Beamer diameter traded vs. transmit power such that 7.1 km array effective diameter capital cost is minimized. 50% wallplug to laser efficiency • Yes, optics cost is low and array diameter is high • 10,000 $/m2 aperture cost (100x higher than baseline) increases CAPEX by 4x and decreases diameter by 4x • $/Watt must fall to keep CAPEX below $10B, but assumed laser cost is still well above current cost of microwaves • Array losses and atmospheric losses yet to be incorporated Parkin Research22 Reference Point Design Example: Trajectory - Circular Dielectric Sail – Reconverged 16 April 2017 100 4.5 1011 4 er 10 w 10 po 10-1 3.5 h ed 9 t r b 10 r y a 3 o g E r s e b m 8 n a ] ] ] o 10 e 2.5 , u fr -2 d a W TJ 10 c i [ [ [ e t t ce e 2 7 n 10 n ec i l a f t K e s i 1.5 r , 6 D -3 10 10 nt e 1 d i 5 c 10 n 0.5 I 10-4 104 0 0.2 1000 1 950 - [ 0.9 η , 900 0.1 m 0.8 ea 850 5 b e r f 0.7 β o tu 800 , a n ] d r o ] c] e i K [ 0.6 t [ ee 0.1 p 750 p m ac S e fr 0.5 T 700 ed ill 0.4 650 0.0 p s 5 n 600 U 0.3 550 0.2 0 500 11000 1 -1 - 10 [ 10000 s t f i 0.9 h 9000 s 5 10-2 er 8000 l 0.9 t n n e o t dopp i 7000 x t ] e a 0.8 ec] r s ed s ’ ] e 6000 t -3 l ar c g 5 l 10 r [ ec a l [ f cce 5000 0.8 e ngu r A A 4000 d n 0.7 10-4 3000 t a n 5 e d 2000 i 0.7 c n 1000 I 0.6 10-5 00 2 4 6 8 10 12 14 16 18 20 5 0 2 4 6 8 10 12 14 16 18 20 0 2 4 6 8 10 12 14 16 18 20 Time in Earth frame [minutes] Time in Earth frame [minutes] Time in Earth frame [minutes] Parkin Research23 Principle coherent phasing methods Single telescope Beam Combining Phased at detector Single telescope Tiled Array Common Optic Amplifiers Individual telescopes Phased at sail Phased Array 24 • Many demos of tiled arrays and Parameter Today Starshot Delta beam combining through a Aperture <1 m ~1 km 103 common aperture. Power 100 kW 100 GW 106 Coherence 3 cm @ 1 kW 3 km @ 1kW 105 • Only one known demo of Length 24 m @ 420 W 125 phasing an array of separated Cost $10/W (large $0.05/W 200 telescopes. quantity scaling) Turbulence Horizontal, Vertical, Starshot distributed, strong, near turbulence branch cuts aperture, easier • Maximum fiber power weak, no demonstrated at required branch cuts Control loop Power in the Cooperative Beacon is non- coherence length is ~400 W. bucket on target coherent trivial beacon Range ~10 km ~ 107 km 106 • Phased arrays for Starshot have significant unsolved phase Target Uncooperative Ultra high Open loop acceleration pointing and sensing and control problems. and speed control TRL 1. 25 26 Return Power vs Range Return signal competes with atmospheric and optics scatter from kW class beam Return signal signal is Doppler shifted to the red ~20% in wavelength at 0.2c Sail Range (m) 27 Path Matching and Phasing Requirement FIBER PHASE NOISE BASE MOTION DISTURBANCES 28 Geometry and coordinate system for the next charts. 29 SAIL ON THE MERIDIAN 30 1 KM ARRAY; OFF MERIDIAN ANGLES 5, 10, 20, 45 DEGREES 31 32 Status of Single Frequency High Power Amplifiers 1064 nm 1 kW ? 420 W 4/17 414 W 1/17 1.2 kW 1/14 33 DISTRIBUTION A Single Frequency PBGF Amplifier 410W MI Regime BKWD SIGNAL ~25 dB Rayleigh SBS B. Pulford, et. at. High Power Narrow Linewidth34 Microstructured Fiber Amplifiers. CLEO 201 High Fill Factor is Required SPARSE ARRAY FILLED ARRAY 35 10 cm subapertures 50 cm subapertures 36 37 1 km array compared to ESO VLT at Paranal Crescent Dunes solar collector 1.25 km diameter 38 Stepped array to allow operation at off zenith angles. 39 Beam Shaping at the Sail is Required One concept for stable beam riding. The beam profile has a potential well in the middle and the spherical sail rides in this well.
Details
-
File Typepdf
-
Upload Time-
-
Content LanguagesEnglish
-
Upload UserAnonymous/Not logged-in
-
File Pages53 Page
-
File Size-