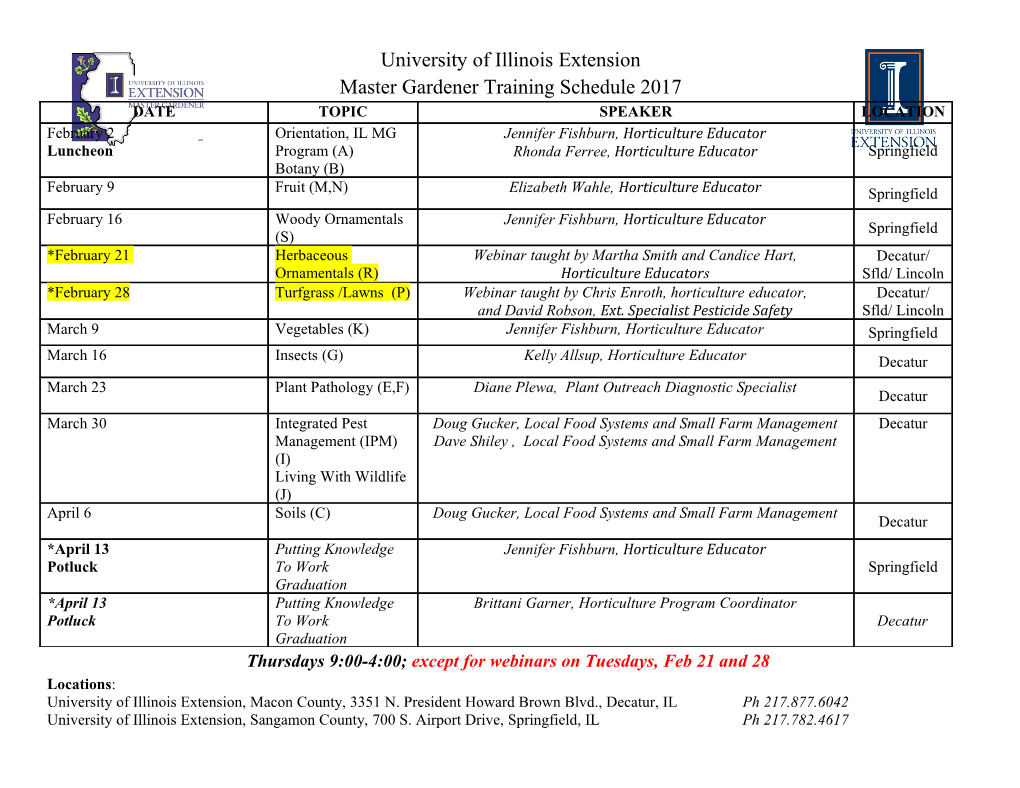
Mechanistic studies of two phosphatase enzymes involved in inostiol metabolism Author: Yang Wei Persistent link: http://hdl.handle.net/2345/3047 This work is posted on eScholarship@BC, Boston College University Libraries. Boston College Electronic Thesis or Dissertation, 2013 Copyright is held by the author, with all rights reserved, unless otherwise noted. Boston College The Graduate School of Arts and Sciences Department of Chemistry MECHANIC STUDIES OF TWO PHOSPHATASE ENZYMES INVOLVED IN INOSITOL METABOLISM a dissertation by YANG WEI submitted in partial fulfillment of the requirements for the degree of Doctor of Philosophy May, 2013 © copyright by YANG WEI 2013 Mechanic studies of two phosphatase enzymes involved in inositol metabolism by Yang Wei Under the direction of Dr. Mary F. Roberts Abstract Inositol-containing molecules and inositol phosphatases have diverse roles in cells. One of the inositol phospholipids phosphatases, PTEN (Phosphatase and Tensin Homolog deleted on Chromosome Ten), is a tumor suppressor and antagonizes the PI3K signaling pathway by dephosphorylating PI(3,4,5)P3 at the 3 position of the inositol ring. In testing predictions of a molecular dynamics simulation, a hydrophobic site adjacent to the active site on PTEN was identified and verified by protein kinetic studies. This hydrophobic site plays an important role in substrate and substrate analogue binding with one of residues, Arg47, critical for PTEN phosphatase activity. Mutations of Arg47 reduced enzyme activities toward both the short-chain substrate as monomers and micelles and long-chain phospholipid presented in vesicles. PI(4,5)P2, the product of PI(3,4,5)P3 dephosphorylation, activates PTEN. Studies by others suggested this occurred when the product was bound to the N-terminal region of the protein (not visible in the crystal structure). However, no direct proof of this existed. The effect of PI(4,5)P2 on PTEN enzyme activities in different substrates systems was studied. 31P NMR was used to probe 31 the spatial location and functional role of PI(4,5)P2 binding site. The fixed field P NMR and high resolution field cycling 31P NMR results indicated there are discrete sites for both substrate and activator lipids on PTEN, and both of sites are spatially separate from the hydrophobic site. The active site, adjacent hydrophobic site, and N-terminal activator binding site worked synergistically to regulate PTEN interacting with the membrane. Thermophilic and hyperthermophilic archaea and bacteria thrive at high temperatures. They often accumulate small organic molecules, called compatible solutes or osmolytes, to protect proteins from thermal denaturation. The thermoprotection mechanism of compatible solutes was explored using inositol monophosphatase (IMPase) from Archaeoglobus fulgidus as the model protein. The protective effect of unusual compatible solutes, di-inositol-1,1’-phosphate (DIP) and diglycerol phosphate (DGP), as well as common compatible solutes glutamate and other anions, on the IMPase thermostability was studied. Specific binding sites of glutamate ions on the IMPase were identified by crystallography and field cycling NMR. However, mutations at these discrete binding sites did not eliminate the thermoprotection, but reduced the thermal stability (Tm) of the protein. Our results indicate the specific binding of osmolytes to the protein exists, but they do not account for the thermoprotection. This dissertation is dedicated to my parents Xiaofei Wei, Lin Yuan and my fiancé Kai Hong i Acknowledgement First, I would like to express my deep gratitude to my advisor, Dr. Mary F. Roberts, for her continuous guidance and support throughout my graduate study. Without her enthusiastic encouragement and confidence, this work would not have been completed. I always appreciate her kindness, patience and generosity. I would like to thank Dr. Goran Krilov and Dr. Qin Wang for doing the simulations of PTEN and discussing this project. I wish to thank Dr. Rebecca Goldstein in the Roberts group for solving the crystal structures of IMPase and collaborations on this project. I wish to thank Dr. Scott Miller, Dr. Yingju Xu and Dr. Christina Longo for providing synthetic deoxy-PI and DIP compounds. I would like to thank Dr. Eranthie Weerapana and Julianne Martell for doing mass spectrometry and data analysis of PTEN spin-labeling samples. I also thank Dr. Weerapana for being my committee member and spending time to read my thesis. I would like to thank Dr. Patrick Wintrode for letting me work in his laboratory to use hydrogen exchange mass spectrometry to study PTEN and PI-PLC. I would like to thank Dr. John Boylan and Dr. Thusitha Jayasundera at Boston College and Dr. Alfred Redfield at Brandeis University for maintaining the NMR facility to make my extensive usage of NMR possible. I wish to thank Dr. Jianmin Gao for letting me use some equipment in his laboratory and being my committee member to read my thesis. ii I wish to thank Dr. Olga Gursky for being a committee member and reading my thesis. I would like to thank Dr. Yanling Karen Wang in the Roberts group for her guidance in my first year. I would like to express my thanks to present and past members of the Roberts group for discussions and help to my projects. I am happy to work and be friends with them. Last, a special thank goes to my family, my parents Xiaofei Wei and Lin Yuan, and my fiancé, Kai Hong, for their love, care and support in my life. iii Abbreviation A. fulgidus Archaeaoglubus fulgidus AHS adjacent hydrophobic site C2 protein kinase C conserved region 2 CD circular dichroism CMC critical micelle concentration CSA chemical shift anisotropy DAG diacylglycerol Dansyl-PE 1,2-dioleoyl-sn-glycero-3-phosphoethanolamine-N-(5- dimethylamino-1-naphthalene-sulfonyl) DGP diglycerol phosphate D-I-1-P D-myo-inositol 1-phosphate diC6PI 1,2-dihexanoyl-sn-glycero-3-phospho-(1’-myo-inositol) 1,2-dihexanoyl-sn-glycero-3-phospho-(1’-myo-inositol-3’,4’- diC6PI(3,4)P2 bisphosphate) 1,2-dihexanoyl-sn-glycero-3-phospho-(1’-myo-inositol-4’,5’- diC6PI(4,5)P2 bisphosphate) diC7PC 1,2-diheptanoyl-sn-glycero-3-phosphocholine diC8PI 1,2-dioctanoyl-sn-glycero-3-phospho-(1’-myo-inositol) diC8PI(3)P 1,2-dioctanoyl-sn-glycero-3-phospho-(1’-myo-inositol-3’- phosphate) 1,2-dioctanoyl-sn-glycero-3-phospho-(1’-myo-inositol-3’,4’- diC8PI(3,4)P2 bisphosphate) 1,2-dioctanoyl-sn-glycero-3-phospho-(1’-myo-inositol-4’,5’- diC8PI(4,5)P2 bisphosphate) iv DIP di-myo-inositol-1,1’ phosphate 1,2-dioleoyl-sn-glycero-3-phospho-(1’-myo-inositol-3’,4’- DOPI(3,4)P2 bisphosphate) 1,2-dioleoyl-sn-glycero-3-phospho-(1’-myo-inositol-4’,5’- DOPI(4,5)P2 bisphosphate) DTT dithioreitol FBP fructose 1,6-bisphosphate G6P glucose-6-phosphate I(1,3,4,5)P4 or IP4 D-myo-inositol-1,3,4,5-tetraphosphate I(1,4,5)P3 or IP3 D-myo-inositol-1,4,5-triphosphate IMPase inositol monophosphatase IP inositol phosphate IPTG isopropyl-β-D-thiogalactopyranoside L-3,5-dd-diC8PI L-3,5-dideoxy-dioctanoyl-phosphatidylinositol L-I-1-P L-myo-inositol 1-phosphate LUV large unilamellar vesicle MTSL S-(2,2,5,5-tetramethyl-2,5-dihydro-1H-pyrrol-3-yl)methyl methanesulfonothioate NBP N-terminal PI(4,5)P2 binding patch NMR nuclear magnetic resonance PA phosphatidic acid PAGE polyacrylamide gel electrophoresis v PCR polymerase chain reaction PDZ post synaptic density protein, drosophila disc large tumor suppressor, and zonula occludens-1 protein PH pleckstrin homology PI phosphatidylinositol PI(3)P phosphatidylinositol 3-phosphate PI(3,4)P2 phosphatidylinositol 3,4-bisphosphate PI(3,4,5)P3 phosphatidylinositol 3,4,5-triphosphate PI(3,5)P2 phosphatidylinositol 3,5-bisphosphate PI(4,5)P2 phosphatidylinositol 4,5-bisphosphate PI3K phosphatidylinositol 3-phosphate kinase PLC phospholipase C POPC 1-palmitoyl-2-oleoyl-sn-glycero-phosphocholine PS phosphatidylserine PTEN phosphatase and tensin homolog deleted on chromosome ten Pyrene-PE 1,2-dioleoyl-sn-glycero-3-phosphoethanolamine-N-(1- pyrenesulfonyl) SDS sodium dodecyl sulfate SUV small unilamellar vesicle TX-100 triton X-100 WT wild type vi Table of Contents Chapter 1 Introduction ................................................................................................... 1 1.1 Inositol and inositol metabolism .............................................................................. 2 1.2 Function of inositol phosphates in eukaryotes ......................................................... 6 1.3 Function of phosphoinositides in eukaryotes ........................................................... 7 1.3.1 Phosphatidylinositol monophosphates: PI(3)P, PI(4)P and PI(5)P ...................... 8 1.3.2 Phosphatidylinositol bisphosphates: PI(3,5)P2, PI(4,5)P2 and PI(3,4)P2 ........... 10 1.3.3 PI(3,4,5)P3 and the PI 3-kinase (PI3K) signaling pathway ................................ 12 1.4 PTEN...................................................................................................................... 15 1.4.1 Substrate specificity ........................................................................................... 16 1.4.2 PTEN structure ................................................................................................... 17 1.4.3 PTEN membrane binding and the role of the N-terminal loop .......................... 21 1.4.4 PTEN C-tail, regulation by phosphorylation...................................................... 27 1.4.5 Regulation of PTEN by other post-translational modification........................... 30 1.4.6 PDZ binding domain and regulation of PTEN by protein-protein
Details
-
File Typepdf
-
Upload Time-
-
Content LanguagesEnglish
-
Upload UserAnonymous/Not logged-in
-
File Pages224 Page
-
File Size-