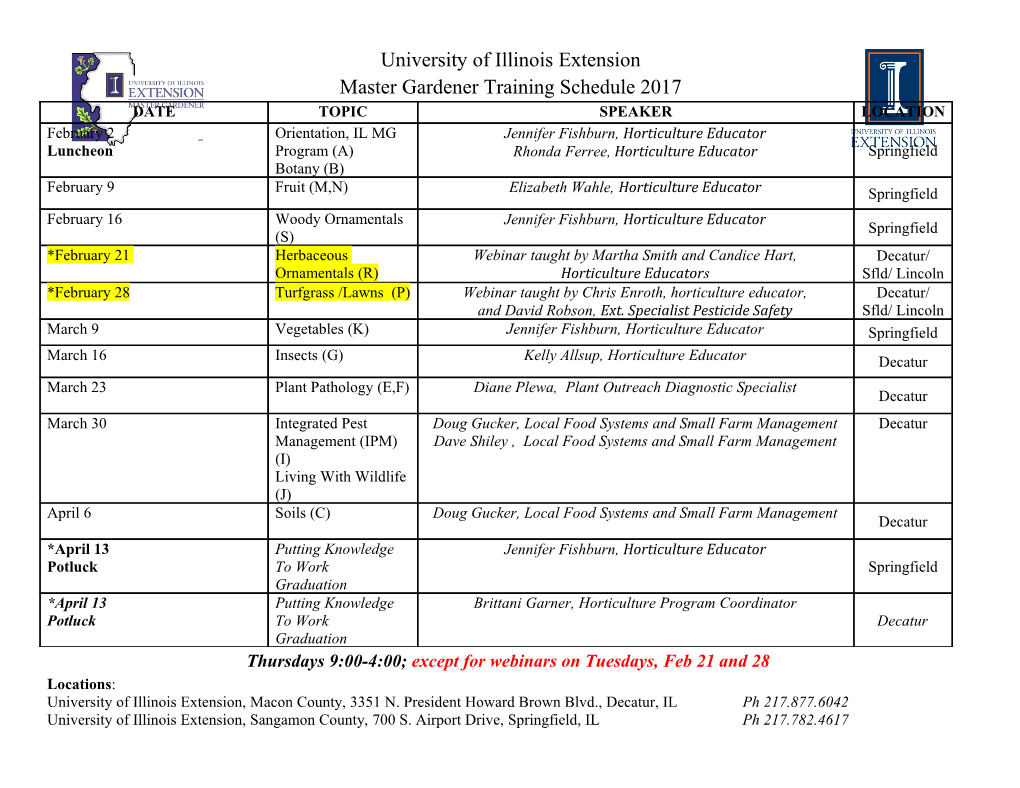
Plasma Physics Reports, Vol. 23, No. 9, 1997, pp. 742–750. From Fizika Plazmy, Vol. 23, No. 9, 1997, pp. 801–810. Original English Text Copyright © 1997 by Kesner, Mauel. MAGNETIC CONFINEMENT SYSTEMS Plasma Confinement in a Levitated Magnetic Dipole J. Kesner* and M. Mauel** * Massachusetts Institute of Technology, Cambridge, Ma 02129 ** Columbia University, New York, N.Y. 10027 Received April 1, 1995 Abstract—Plasma confinement in the field of a levitated dipole offers many advantages for magnetic fusion. MHD stability is obtained from compressibility which utilizes the large flux tube expansion of a dipole field. Such a device could be high beta, steady state, and exhibit good confinement properties. The large flux expan- sion will ease the difficulty of the divertor design. The configuration is ideal for electron cyclotron heating and controlled convective flow patterns may provide a mechanism for fueling and ash removal. 1. INTRODUCTION tribution function, µ is the adiabatic invariant, µ = The dipole magnetic field is the simplest and most e⊥/2B, J is the parallel invariant, J = °∫v || dl and the flux, common magnetic field configuration in the universe. It ψ is the third adiabatic invariant. The frequencies that is the magnetic far-field of a single, circular current Ω correspond to these invariants are respectively c the loop, and it represents the dominate structure of the cyclotron frequency, ω , the bounce frequency and ω middle magnetospheres of magnetized planets and neu- b d tron stars. The use of a dipole magnetic field generated the curvature driven precessional drift frequency. Both by a levitated ring to confine a hot plasma for fusion of these conditions lead to dipole pressure profiles that scale with radius as r–20/3 while the adiabatic distribu- power generation was first considered by Akira Haseg- µ awa after participating in the Voyager 2 encounter with tion function, F( , J) also implies a density dependence ∝ –4 ∝ –8/3 Uranus [1]. Hasegawa recognized that the inward diffu- of ne r and a temperature dependence T r . sion and adiabatic heating that accompanied strong By levitating the dipole magnet in order to prevent magnetic and electric fluctuations in planetary mag- end losses, conceptual reactor studies supported the netospheres represented a fundamental property of possibility of a dipole fusion reactor [3, 4]. The dipole strongly magnetized plasmas not yet observed in labo- reactor concept is a radical departure from the better ratory fusion experiments. For example, it is well- known toroidal-based magnetic fusion reactor con- known that global fluctuations excited in laboratory cepts. For example, the most difficult problems for a fusion plasmas result in rapid plasma and energy loss. tokamak reactor are the divertor heat dissipation, dis- In contrast, large-scale fluctuations induced by sudden ruptions, steady state operation, and an inherently low compressions of the geomagnetic cavity (due to beta limit. Furthermore, the tokamak is subject to neo- enhancements in solar wind pressure) or by unsteady classical effects and micro-turbulence driven transport. convections occurring during magnetic substorms The dipole concept provides a approach to fusion energize and populate the energetic electrons trapped in which solves these problems. the Earth’s magnetosphere [2]. The fluctuations induce inward particle diffusion from the magnetospheric 1. Divertor problem: The difficulty in spreading the boundary even when the central plasma density greatly heat load at the divertor plate is generic to concepts in exceeds the density at the edge. Hasegawa postulated which the magnetic flux is trapped within the coil sys- that if a hot plasma having pressure profiles similar to tem. By having the plasma outside of the confining coil those observed in nature could be confined by a labora- the magnetic flux can be sufficiently expanded to sub- tory dipole magnetic field, this plasma might also be stantially reduce divertor heat loads. immune to anomalous (outward) transport of plasma energy and particles. 2. Major disruptions: A tokamak has a large amount of energy stored in the plasma current. The dipole The dipole reactor concept is based on the idea of plasma carries only diamagnetic current and is inher- generating pressure profiles near marginal stability for ently free of disruptions. Experiments observe relax- low-frequency magnetic and electrostatic fluctuations. ation oscillations, reminiscent of tokamak ELMs, From ideal MHD, marginal stability results when the which relax the pressure gradients when they become pressure profile, p satisfies the adiabaticity condition, too large. δ(pVγ) = 0, where V is the flux tube volume and γ = 5/3. From gyro kinetics, marginal stability results when 3. Steady state: A tokamak is a pulsed device and ∂F(µ, J, ψ)/∂ψ = 0 where F(µ, J, ψ) is the particle dis- current drive schemes that are required for steady oper- 1063-780X/97/2309-0742 $10.00 © 1997 åÄàä ç‡Û͇ /Interperiodica Publishing PLASMA CONFINEMENT IN A LEVITATED MAGNETIC DIPOLE 743 ation appear to be costly. The dipole plasma is inher- a substantially improved approach to magnetic fusion ently steady state. energy production. 4. Beta limit: Tokamak stability depends on the poloidal field which is less than the toroidal field by 2. DIPOLE CONFIGURATION Bp/BT ~ 1/qA with q the edge safety factor and A the aspect ratio. For a dipole there is a critical pressure gra- Figure 1 which shows the magnetic flux contours dient that can be supported (due to the compressibility and the mod-B surfaces for a laboratory-size levitated term in the interchange stability criterion) and for a suf- dipole facility [11]. The levitated ring has a major ficiently gentle pressure gradient the dipole plasma radius Rc and a minor radius a. The simplest vacuum resides in an absolute energy well and is stable up to chamber geometry would be a spherical tank and the local beta values in excess of unity. last closed flux surface will be assumed to have a radius ≥ 5. Transport and neoclassical effects: The trapping Rw. Typically Rw 5Rc to permit a substantial reduction of particles in regions of bad curvature makes the toka- of plasma pressure. The plasma pressure will attain a mak susceptible to drift frequency range trapped parti- peak value at a midplane radius of R0. Between the ring cle driven turbulent transport. A dipole could, theoreti- surface (located at R = Rc + a) and the peak pressure cally, show classical transport. In addition a tokamak location there is good curvature and since the field is has a “neoclassical” degradation of transport that relatively high near the ring, cross-field transport is be derives from the drifts of particles off of the flux sur- expected to be low. Thus we expect high pressure gra- faces. In a dipole the drifts are toroidal and they define dients in this region. The schematic midplane pressure the flux surfaces. profile is shown in Fig. 2. The chief drawback of the dipole approach is the need for a levitated superconducting ring internal to the plasma. Although this provides a challenge to the engi- Z, Òm neering of the device recent advances in high tempera- –300 ture superconductors coupled with an innovative design concept of Dawson [5, 6] on the maintenance of an 5 × 10 internal superconducting ring in the vicinity of a fusion –3 plasma leads to the conclusion that this issue is techno- 1 × logically solvable. –200 10 –2 Utilization of electron cyclotron resonance heating (ECRH) and pellet injection in a levitated dipole per- mits a unique heating approach for the creation of fusion grade plasmas. The combination of closed field –100 lines and high beta makes the dipole an ideal geometry for creating a high beta, hot electron plasma by the application of ECRH. Such plasmas have been demon- strated in open field line mirror machines as well as in 2 × levitrons and in the non-levitated dipole (or so-called 0 10 “terrella”) CTX experiment [7–10]. The density of the –1 5 5 10 hot electron plasma is observed to be limited by micro- × wave accessibility and the energy by relativistic detun- –1 1 1 × ing. Unlike ECRH mirror applications, the hot elec- 10 trons created in a levitated dipole can only be lost by 100 –1 cross-field transport and if transport is close to classical the resulting loss rate will be small. The energy stored in the hot electron plasma can be transferred into a dense, thermal hydrogenic plasma by injecting pellets into the ECR heated plasma. 200 We conclude that the continued advances in the understanding of magnetospheric plasmas coupled with the development of the technology of gyrotrons and of pellet injectors provides the fusion community 300 with a new and unique approach to creating fusion 0 100 200 300 400 grade laboratory plasmas. In addition the development R, Òm of high temperature superconductors and of new con- ceptual approaches to the cooling of an internal levi- Fig. 1. Magnetic field and flux surfaces for a levitated dipole tated superconducting coil provide a potential path for experiment. PLASMA PHYSICS REPORTS Vol. 23 No. 9 1997 744 KESNER, MAUEL p(R) The vacuum field of a circular loop provides a useful analytic approximation. The vector potential for the field of a single loop of radius Rc in cylindrical coordi- nates has the form 12⁄ µI R c 2 2 2 Aθ =------ ----- []()1– 0.5k Kk()–Ek(),(1) πkr Rc R0 Rw R with B = ∇ × A and the magnetic flux surfaces are given by rAθ. K and E are complete elliptic integrals of the Fig. 2. Midplane pressure profile shown schematically. first and second kind. The ring current is I and 4Rr k2= -------------------------------c- . Outside of the peak pressure region the pressure will ()R+r2+z2 decay in a region of “bad” curvature and MHD stability c requires a relatively gentle pressure gradient.
Details
-
File Typepdf
-
Upload Time-
-
Content LanguagesEnglish
-
Upload UserAnonymous/Not logged-in
-
File Pages9 Page
-
File Size-