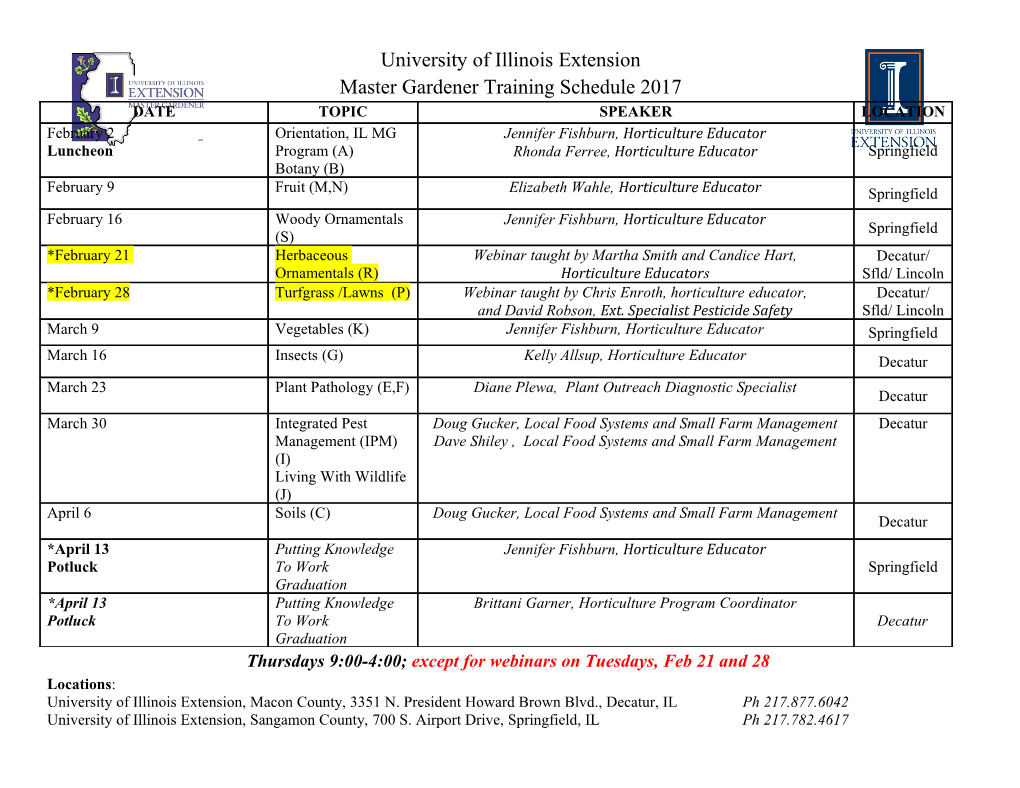
Vg)I,. 78, NO. 26 •[OURNAL OF GEOPHYSICAL RESEARCH SEPTEMBER 10, 1973 Shock-InducedTransition of Quartzto Stishovite JOHN D. KLEEMAN 2 AND THOMAS J. AHRENS SeismologicalLaboratory, California Institute o• Technology Pasadena, California 91109 The transformation of quartz to stishovite has been studied by X-ray and optical exami- nation of a series of experimentally shock-loaded specimens of a quartz-copper mixture. Shock pressuresof 68 to 260 kb and peak temperatures of 320ø to 870øK were achieved. Stishovite was identified from quartz shock-loaded above 90 kb; the quantity increases with increasingpressure, but is not dependent on temperature. The formation of stishovite under shock conditionsappears to be intimately related to a short-range order phase. Stishovite is a high-pressurepolymorph of and Rosenberg [1968] indicate an increasing silicon dioxide, having the same structure as proportionof high-pressurepolymorphs above rutfie and cassiterite. It is found in nature as a 120 kb. DeCarli and Milton [1965] suggested minute componentof shockedquar•tz-bearing that the major proportionof stishoviteat equi- rocks associatedwith meteorite impact. Stisho,v librium reverts to an amorphous, or short- and PoTova [1961] first produced this phase range order (SRO), phase upon releasefrom in static high-pressure experiments. Shortly the high-pressureshock state. Our first experi- afterward, Chao et al. [1962] found small quan- ments revealedno significantquantities of SRO tities of stishovite in the intensely shocked phase coexistingwith the minute fraction of quartz sandstoneat. Meteor Crater, Arizona. stishovite recovered from shock pressureso,f Subsequentwork [Chao and Littler, 1963] has about 180 kb. We wish to characterize the demonstrated the natural occurrence of this occurrenceof SRO phasein relationto stisho- mineral at only one other impact crater (Ries vite formation. basin, Germany). In 1965 stishovite was first The third objectiveof this study was to in- isolated from experimentally shocked quartz vestigatethe parameterslikely to reflecton the [DeCarli and Milton, 1965], although it was quantity of stisho,viteproduced. Factors such not possibleto compare,in detail, the pressure as temperature (both in equilibrium shock and temperatureconditions required to produce state and in postshockstate), rate of cooling stishovite dynamically with the static results. duringshock pressure release, duration of shock, One impo•ant objectiveof the present study as well as the amount of pressureoverdriving, was to determine the lowest shock pressure were thought to be potentially effective in required to produce stishovite from quartz. varyingthe yield of recoveredstishovite. The Measurementso,f the Hugoniot equation of resultsof this aspectof the study will help in state of quartz indicate that stishovite,with or selectingthe optimumconditions for producing without coesite,will form a major part of the other high-pressureminerals in the laboratory. phase assemblageat pressuresabove 140 kb BAS•S OF EXPERIMENTS [Wackerle,1962; McQueenet al., 1963]. Ahrens The impactof a metal flyer plate,imbedded at the front of a Lexan plastic projectile,in- x Contribution 2203, Division of Geological and ducesa shockwave in a targetassembly (Figure Planetaw Sciences,California Institute of Tech- 1). The shockstate producedin the target is nology, Pasadena, California. determinedfrom the measuredflyer-plate veloc- •Permanent address: Department of Geology, University of New England, Armidale, N.S.W., ity and the Itugoniotsof the targetand flyer- Australia. platematerials by theimpedance match method [Rice eta/., 1'958].In theseexperiments the Copyright ¸ 1973 by the American GeophysicalUnion. sampleconsisted of a mixtureof quartzand 5954 KLEEMANAND AHRENS' QUARTZTO STISHO¾ITE 5955 copperpowders (quartz, 6% by weight). The Hugoniots for tungsten alloy (the usual pressure-volumeHugoniot for the mixture (as- striker plate material' 16.8 g/cmS), the copper- sumed to retain no porosity) was constructed quartz aggregate, and the stainlesssteel encas- fro.m the data for the individual components ing the sample are shown schematically in usingthe relation [McQueen et al., 1970] Figure 2. Experimental data for constructing 2 the Hugoniots shown in Figure 2 are taken V(p) = • m•V•(p) (1) from McQueen et al. [1970], Ahrens and Ga#- i--1 hey [1971], and Wackerle [1962]. where V{ is the specificvolume of the ith com- The duration of the shock pulse was deter- ponentat pressurep and m{ is the massfraction mined by the time taken for the rarefaction of this component.The assumption,implicit in originating from the rear of the striker plate Figure 2, is that the quartz and copper are to pass the same point as the shock front. By both shocked to pressure-volumestates along calculating the velocities of shock wave and their individual Hugoniotscentered at standard rarefaction, a time-distanceplot can be con- conditions.However, the internal energy in- structedfor one geometry[Gibbons and Ahrens, crease of the mixture depends upon initial 1971]. For the 5-mm thick flyer plates used in porosity. Before shocking, the mixture was these experiments, the shock pulse duration is compactedinto a hard pellet usinga reproduci- about 2 ysec.There is also a reflectedcompres- ble pressure.The density of the compressed sion originating from the back of the sample material is also reproducible.Since the sample cavity, from the nonporous denser stainless was porous before shock loading, the appro- steel. This reflection will enhancethe pressure priate Hugoniot for use in the impedance in the rear of the sample cavity, and fo•; this match method was calculated from the relation reason no quartz was mixed in the copper powder in this volume (see Figure 1). There u = [p(r• - r)] •/2 (2) shouldnot be a significantpressure gradient in where u is the particle velocity of the mixture, the quartz-copper portion of the target. V• is the specificvolume of the porousmixture The use of coppermixtures has somedistinct at zero pressure,and V is the specificvolume advantages.The Hugoniot of the sample mix- ture is closer to that of stainless steel than at pressure p, as calculated from Figure 2. Equation 2 amountsto the 'ideal locking' model quartz (see Figure 2), especially when com- of Linde and Schmidt [1966]. pared with porous quartz aggregates (e.g., pressedquartz powder or sandstone).This re- NICHROME sults in lessperturbation of the shock front as THERMOCOUPLE LEADS F HEATINGELEMENT it passesthrough the sample,giving more even / SAUREISENCEICERAMIC"7 distributionO f pressure,especially at the edges, o-•o •ooooooo o r and more favorable conditions for recovery intact. In addition, higher pressurescan be CAP obtained with the same projectile velocity. An- other important advantage is temperature con- trol. Since the thermal equation of state of copper is well determined [McQueen et. al., 1970], the shocktemperature can be calculated. LEXAN PROJECTILE The postshock temperature achieved in the 3 0 0 0 0 ) 0 0 0 SiO• experimentsis largely controlled by the STRIKER PLATE- propertiesof the copper.In addition,the copper STEEL conductsheat rapidly into the stainlesssteel block, which acts as a heat sink, quicklyreduc- • QUARTZ-COPPERMIXTURE ing postshocktemperatures. •. COPPERALONE SHOCK AND POSTSHOCK TEMPERATURES Fig. 1. Diagrammatic cross section of the shock recovery experiment. Projectile velocity and The copper-quartzmixture is relatively in- thermocouple voltage are recorded before impact. compressible,and shockpressures are relatively 5956 KLEEMANAND AHRENS' QUARTZTO STISHOVITE modest,so that the entropyproduction is not great, and the shock and postshocktempera- tures are largely controlledby the preshock temperature. Nevertheless,it is useful to at- tempt to approximately calculate shock and postshocktemperatures for these experiments. If, upon shock compressionthe quartz frag- ments remain at their initial maximumgrain size of 0.01 to 0.025 cm in diameter, thermal equilibriumwith the surrounding copper matrix takes place in the order of 10-5 sec. Since the high-pressureshock duration is 2 x 10-6 sec, onlythermal equilibrium in the postshock state will be achievedwithin the copper matrix. On the other hand, if a significantnumber of quartz grainsare crushedby the shockand the effective particle size is significantly reduced, thermal equilibrium (largely controlled by the shock state in the copper) shouldbe achievedduring passageof the stress wave. For the present (Uo,Po) calculation,the latter case is assumed.This 0 0.5 1.0 1.5 assumption is not critical, as the postshock P(:]rf•cle velocity, km/sec and shock temperatures are nearly equivalent, Fig. 2. Pressure-particlevelocity plane indi- sincethe shock-inducedtemperature rise is caringshock state (u•, px) produced by impact largely accountedfor by the irreversiblework of flyer plate with velocityuo into stainlesssteel done on the sample in crushingout initial cap. Transmittedstress wave producesshock porosity. statein copper-quartzmixture (p•, u•). Two estimatesof shockand postshocktem- peratureswere obtained. Asa firstapproxima- ing the entropy increases [Ahrens, 1972] for tion,the actual quartz content ofthe samples the two cases outlined above and comparing wasneglected. A lower temperature wascalcu- the entropies with thermodynamic tabulation latedby assumingthat the samples were en- of entropyversus temperature at standard tirelycopper and the density (6.45 to 6.55 pressure[Robie and Waldbaum, 1968], it was g/cm3) arosewholly from
Details
-
File Typepdf
-
Upload Time-
-
Content LanguagesEnglish
-
Upload UserAnonymous/Not logged-in
-
File Pages7 Page
-
File Size-